Can you tell us a bit about your background?
I began my dive into genetics and aquaculture a few years after I had arrived at college. I’ve always been fascinated by nature, specifically the ocean. As many of you might agree, being too far from the sea brings with it a sense of… claustrophobia. I grew up outside of Boston in a small farm town where I worked on the fields during summers. Food has therefore always been important to me. Food and a fascination with the wonders of the natural world drew my family and I on trips all across the world. Trips I always came back from with a deeper sense of global understanding. With my curiosity about biology, scientific mind and entrepreneurial spirit, I set off to college to learn more about genetics and biotechnology, and to learn what exactly I could do with this passion.
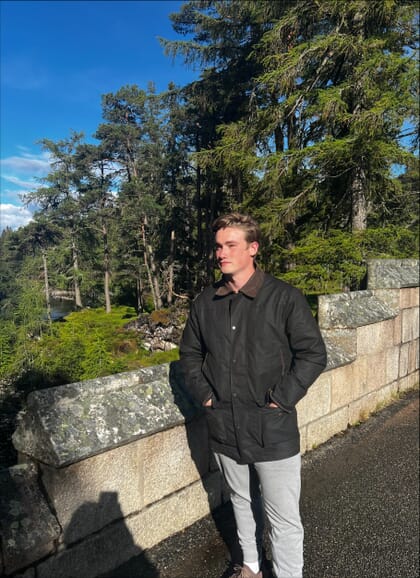
Sibley has a long-standing fascination with biotechnology, genetics and all things aquatic
What/where are you studying at the moment?
At present, I’m a senior undergraduate at Northeastern University, in Boston. My academic focus has been on biotechnology and aquaculture, fields that may have been quite distinct a decade ago, but are increasingly intertwining. Northeastern University is widely known for its co-op programme, which requires students to pursue their academic interests on six month internships wherever we desire. Since 2019, I’ve had three co-ops – two with pharmaceutical biotechnology companies and one in Boston’s seafood preparation and distribution scene. This May I will be graduating with a bachelors of science in cell and molecular biology and a minor in entrepreneurial startups, a crossover that has allowed me to explore the hard sciences behind emerging biotechnologies and to discover how I might apply this science in the real world.
What inspired you to sign up to a genomics module?
Given my distinct major and minor requirements, I haven’t had much of an opportunity to properly explore my deepest interests through scientific electives. With this semester being my last, I’ve finally had the chance to design my course load with the full freedom of Northeastern’s hundreds of offerings. So I enrolled in two advanced sciences: genome editing, and stem cells and regeneration. Meanwhile my own self-directed undergraduate dissertation relates to applied genome editing in aquaculture. My inspiration for this was simple – gene editing is on the cusp of transforming the world and its application will be led by those both curious and knowledgeable enough to do so. CRISPR technologies have entered a “sink or swim” phase of explosive utilisation, and I plan to do the latter.
Speculating about the applications of CRISPR gene-editing technology is surely exciting and inquisitive research about how we might do so is what inevitably drives further discovery and development. It is important to remember however, that fundamental research drives technological advancement. It often seems as though science leads to empty promises, while the reality is more that speculated implementation is more difficult than an eye-catching headline might imply. Understanding biological systems drives their derived technological advancements, as CRISPR has shown. It will be the continued pursuit of knowledge and the unravelling of nature’s mechanisms that will allow us to progress with genome editing technologies, a fundamental truth that must not be forgotten as we ideate with newfound capabilities. At the end of the day, how well the road beneath a car is built limits how fast you can drive, not the engine.
Why do you think that aquaculture is particularly ripe for the use of gene editing?
Applied gene editing with CRISPR tools relies heavily on the foundational knowledge of the organism you might be trying to edit. As of 2021, 594 fish genomes had been sequenced, including most popular farmed species. De novo
sequencing, or sequencing the entire genome of a new species for our purposes, can now be done faster than ever before. With this comes successive research on how newly discovered genes are expressed by the fish. As databases of genomic information are methodically developed, our ability to edit genes and their expression becomes quite real, now more than ever with CRISPR. Given the vast and ever-growing genomic libraries we have today in regards to farmed species and the continuous innovations in CRISPR tools to provide more gene editing capabilities, the realistic application of CRISPR-edited fish is finally upon us.
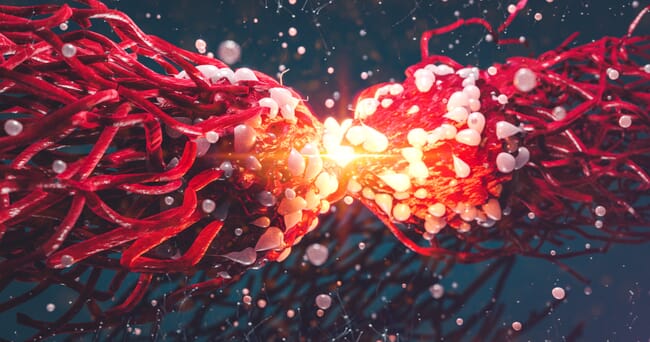
CRISPR technology uses Cas proteins to identify and make precise edits specific DNA fragments
What is the basis of CRISPR?
CRISPR itself is an acronym for clustered regularly interspaced short palindromic repeats – which is a mouthful. It refers to genetic fragments left behind in a bacterial genome after multiple infections from a specific form of virus. Bacteria get viral infections just as we do. One type of bacterial virus, known as bacteriophages. Bacteriophages insert their DNA into the bacteria, which is then translated by the bacteria much like how it reads its own genome. Bacteriophages have devised a method in which they replicate only by tricking bacterial cell machinery. A technique so effective, they have evolutionarily “streamlined” beyond any capability to reproduce on their own they likely once had. It’s quite an interesting, albeit destructive, dependence.
In the end, an infected bacterium ends up building more and more bacteriophages within itself until it bursts open, spreading them elsewhere. You can see how impressive this infectious mechanism is. One infected bacterium might build great sums of bacteriophages before bursting. Evolutionarily, bacteria had to design a form of defence against bacteriophages, lest they become nothing more than virus factories.
In recent years, one mechanism in particular was discovered regarding how bacteria defend themselves against invading bacteriophages. Many bacteria possess Cas proteins, which are naturally engineered to seek out specific foreign genomic information. Let’s say a Cas protein evolved within a bacterium to defend itself against bacteriophage X. If virus X infects the bacterium with its unique DNA, the Cas proteins will act to cut the invading DNA into useless fragments. Not only this, but the Cas proteins may return small, harmless fragments of virus X’s DNA (called spacers) to the bacterium’s genome, so that it might better identify virus X in the future and respond more quickly and precisely. Furthermore, since the bacterium’s DNA is passed down through the generations, future bacteria will have the same defence. This is what CRISPR is, DNA fragments in the bacteria’s genome that help it defend itself against bacteriophages – acquired over time.
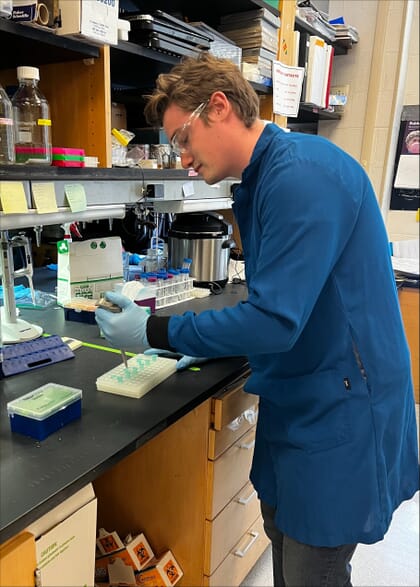
Cas variants have already been designed to disable genes, overexpress genes, insert new genes and even reconfigure entire chromosomes
You can imagine the accuracy and precision with which CRISPR-Cas systems must operate. The slightest imprecision and a Cas protein might destroy the bacterial genome. Now, combine all of the traits described here about the CRISPR-Cas system and you can begin to see why geneticists are so incredibly excited about it.
- Cas proteins can make incisions in DNA at specific targets. Precision.
- Cas proteins can insert new genetic information into a genome. Editing.
- Cas proteins are programmable. Tractability.
How has the tech been evolving?
CRISPR editing tools have only been utilised since the 2010s. Yet the rate of discovery and advancement has been astronomical. Researchers have discovered and designed new Cas protein variants to do their bidding. Variations of Cas work across most organisms. It’s simply a programmed protein, after all.
Cas variants have already been designed to disable genes, overexpress genes, insert new genes and even reconfigure entire chromosomes. There is even a Cas editing strategy employed to change nucleotides without even making an incision in the DNA. There is much more I could cover here, but in summary CRISPR has fundamentally changed our ability to control genes and their expression.
Today, CRISPR is no longer just used in research. Gene therapies have been designed which utilise programmed Cas proteins to cure patients with previously-incurable genetic disorders. CRISPR-edited crops are already being grown in countries that permit it such as China and Ecuador, and research continues in those that yet don’t. CRISPR has come so far as to bring into question an edited organism’s legal status as a GMO, since CRISPR can be used to replicate precisely how selective breeding or natural mutations might change gene expression over a millennium.
Unsurprisingly, utilising CRISPR requires a host of technologies, computational power and highly skilled scientists. The existing CRISPR gene therapies approved for humans typically cost over $1 million. Prices will undoubtedly come down as competition rises and the underlying infrastructure and supply chains strengthen. The fact that CRISPR therapies even exist already suggests the technology’s awesome potential, and with advancements progressing at the rate that they are, costs will surely come down shortly.
What farmed aquatic species do you think would benefit the most from gene editing?
Considering the breadth and depth with which CRISPR can be applied, most species can have their genome edited with the right supporting information. Therefore, it would be best to begin with species we already have extensive genomic data on. In fact, this has already begun. Researchers at the Norwegian Institute of Marine Research successfully edited Atlantic salmon in 2022 to produce a sterile variant, without the premature maturation which plagued pre-CRISPR sterilisation via triploidisation attempts. This research team says they are also exploring areas such as disease resistance, robustness and omega-3 fatty acid metabolism. Besides salmon, research is underway with CRISPR on tilapia, zebrafish and medaka, most notably. All ongoing CRISPR efforts on farmable fish seek to mitigate problems the industry faces and to enhance the fish beyond its natural capabilities – ranging from eliminating the ecological impact from escaped fish or increasing omega-3 absorption.
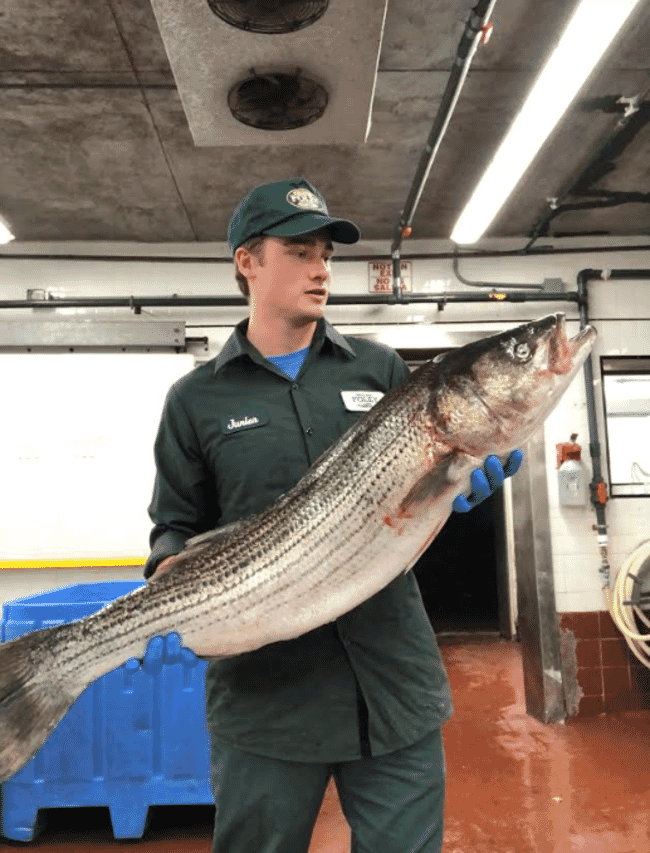
CRISPR shows great promise in innovating the industry's approach to nutrient uptake, fish growth, biosecurity and adaptability
Are there particular traits within these species that tools such as CRISPR would be most suited to improve?
Thinking a bit further into the future, you can imagine just how impactful CRISPR-edited fish might be. Let’s expand upon some of the Norwegian Institute’s research topics by exploring just how these might play out in time:
- Nutrient uptake and expression. One of the greatest challenges in aquaculture today is feed. Salmon feeds, for instance, are typically meticulously designed from scores of ingredients in order to best mimic wild feeding habits and to produce the most nutritious fillets. What if, however, salmon were less dependent on their specific nutrients for optimal health? Numerous fish have significantly less intense dietary requirements than salmon. Combining a versatility enhancement of this nature with the concept of optimising omega-3 absorption could result in healthier fish than we’ve ever seen, with feed costs – both environmental and financial – exponentially reduced.
- Hardiness and environmental adaptability. The world’s oceans, vast as they are, are largely inhospitable for many farmed fish throughout certain stages of their lives. There’s a reason Florida’s largest salmon farm is indoors. The fact that every inch of the ocean has some sort of native fish suggests that environmental adaptability comes down to genetics, as most things do. Salmon variants could be optimised for farming from the deadly currents of the Southern Ocean to the stillest inlets of the tropics.
- Kill switches. Making fish sterile is a great step towards reducing and regulating aquaculture’s impact on the ocean’s ecosystems. If we are to continue down the path of designing our own animals, it would be important to install measures beyond intentional sterility. It would be entirely feasible with today’s CRISPR technology to remove a fish’s ability to create one of its many essential proteins. Herein you can see the “kill switch” – in that a farm could include the essential nutrient in its feed. Morbid? Perhaps, but preventing farmed fish from living more than a week beyond the cages of a farm would prove invaluable in the fight to protect natural ecosystems in the event of an escape.
- Growth rate. Not a novel idea, but the precision with which CRISPR allows genomic editing provides researchers with the ability to enhance growth rates like never before. AquaBounty nearly doubled Atlantic salmon’s growth rate with classical gene editing. Imagine what could be done with CRISPR? Any unwanted side-effects can be ruled out and, unlike modern growth-enhanced livestock, editing with CRISPR will allow there to be no downsides for the fish.
- Inshore and offshore farming of genetically modified fish. Compiling all of the augmentation I’ve listed above will mean nothing if these CRISPR fish can’t be farmed at scale safely. Luckily, the precision of CRISPR strikes yet again. Currently, no genetically engineered fish are farmed off land due to the great concern of unknowns. CRISPR eliminates these unknowns, and will actually provide us with more control than even wild fisheries can ensure. There will soon be no unknowns when it comes to CRISPR editing, beyond the random entropic mutations that all living cells incur naturally. Legislation will soon come around.
Are there any projects in aquaculture – past or present – using CRISPR that have been inspirational for you?
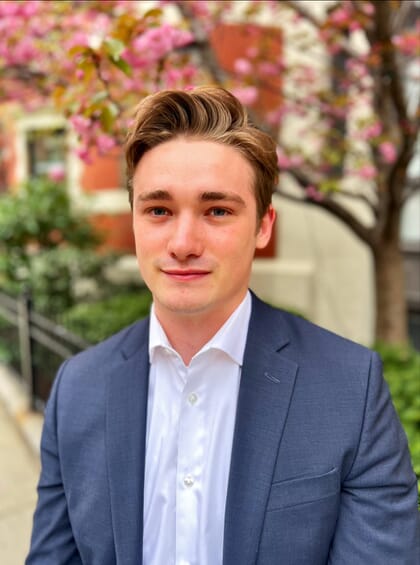
His qualification has allowed him to explore the hard sciences behind emerging biotechnologies and to discover how this science could be applied in the real world
The Norwegian Institute of Marine Research has been working with CRISPR on salmon since 2013, and with their recent publication on sterile and otherwise healthy fish, their goals seem much closer than previously expected. Their team’s dedication to applying CRISPR cautiously and for the good of us all has been a great motivational sight. While I’m not involved with the institute myself, I stay up-to-date with their findings. The team seems to be more excited and knowledgeable about CRISPR technologies than almost anyone, and their holistic purpose should inspire us all to dive into the world of CRISPR.
What are the major barriers – ethical, regulatory and practical – that are preventing CRISPR being more widely applied in commercial aquaculture?
With the possibility to use CRISPR correctly comes the inevitable possibility to use it incorrectly. Since the technology’s inception, researchers have been extremely cautious in an effort to prevent unintentional harm. Gene therapies only make their way into humans if they are upheld to the highest levels of scrutiny, and the same should continue to apply to aquaculture. To address the often raised concern of “just because we can doesn’t mean we should” – we very much should. Billions of lives are at stake as we approach a world with 10 billion mouths to feed on a planet capable of naturally supporting no more than 2 billion.
There is not, nor will there ever be, an absolute guarantee that applied CRISPR technologies will always be safe. No such promise exists with anything. And yet, it’s hard not to be wary of gene editing without such a guarantee. This unease is reflected in today’s legislation. Most governments classify CRISPR technology alongside its classical gene editing predecessor, and therefore it will be some time before CRISPR-edited fish are farmed in open waters. Awareness campaigns and large industry movements are already underway to open the door to CRISPR, legislatively. It’s a matter of conveying the magnitude of difference between classical gene editing techniques and CRISPR to the public in an appreciable way.
The practical barriers to applying CRISPR in aquaculture commercially should not be too surprising. Legislation, funding, global cooperation and public education are likely the most important hurdles to overcome. Many more nuanced practicalities will hinder CRISPR’s full utilisation at first, but with a technology this groundbreaking, nothing and no one can or should stop it. Cooperation is key, and with it and CRISPR we might just save the planet and ourselves in time.
What are your plans after you graduate and what would you like to be doing in 10 years’ time?
I wish I had a definitive answer here. After I graduate, I will begin looking towards my post-graduate education while I find a position in the industry that will best suit my upward development in the field. Some of the best universities and institutions are scattered across the globe, and I truly hope to learn under the best, wherever that might be. With my focus on genetics and aquaculture, I hope to join the industry with as much impact as I can muster. Only time will tell, but I wholeheartedly believe the applications of CRISPR reach far beyond healing ourselves. We can heal our planet, our food system and ourselves simultaneously with applied CRISPR technologies, and that is what I hope to be doing in 10 years’ time.