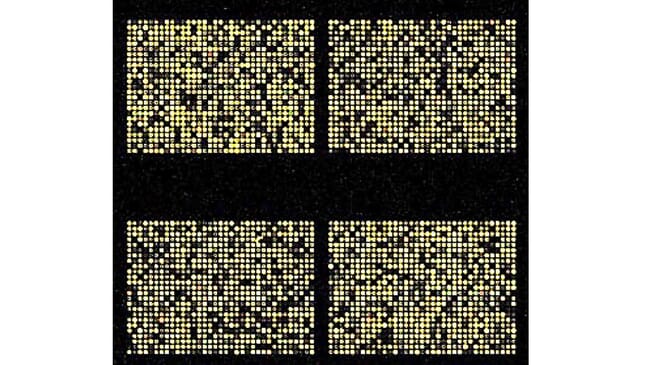
In a recent review of CRISPR technology in this column, it was clear that the technique has not yet contributed significantly to improving disease issues in aquatic organisms. But there is another emerging molecular approach that is already beginning to provide significant advances in pathogen resistance in many aquaculture species. Genomic selection relies on an analysis of many hundreds or thousands of genes throughout the genome (the entire genetic material present) to develop an overall genetic profile for an individual organism, with the highest scoring individuals reserved for breeding purposes.
While this approach to selective breeding is considered cutting-edge in aquaculture, the practice of selection has always been about developing the best estimate possible of an individual animal’s (or plant’s) genetic worth. For centuries (well… decades, when we talk about aquaculture) selection progress was reliant on what we could observe and measure, and the extent to which superior performance was inherited. Eventually, some improvement in selection efficiency was obtained by also taking into account observations and measurements from relatives of potential breeding candidates. But breeders’ choices were still dependent on observations and measurements.
As methods of mapping genes on chromosomes and determining which alleles were present at specific genes advanced rapidly in the 1980s and 1990s, the concept of quantitative trait loci (QTLs) emerged. Using molecular markers to identify the locations of genes that had comparatively significant effects on traits of interest (QTLs), researchers were able to evaluate the location and influence of these genes of importance.
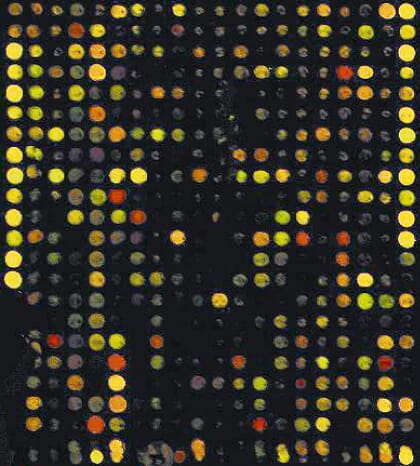
One of the earlier reports of QTL evaluation in aquaculture involved a study of genetic influences over upper temperature tolerance in rainbow trout. There was a significant association between upper temperature tolerance and the alleles present at two specific markers. The QTLs inferred from the results accounted for roughly 13 percent and 9 percent of the overall additive (heritable) variation for the trait, and several other markers appeared to have some association as well. Similar studies were undertaken with a number of species and traits of interest.
But QTLs could only explain some limited portion of the genetic control within a population. This is because quantitative (normally distributed) traits are generally controlled by large numbers of distinct genes, many of which have only small impacts when evaluated individually. QTLs were helpful in improving production traits in some aquatic species, but in most cases not really a game-changer.
The idea of looking at entire genomes of plants and animals was already being considered at the time QTLs became popular. Remember the Human Genome Project? Researchers sequenced some 30,000 genes, literally transcribing the natural order of the basic building blocks of these structures (individual nucleic acids) and identifying variations that occur within or among individuals. Humans have 23 pairs of chromosomes, and researchers have determined that each chromosome contains 50 million to 300 million base pairs of nucleic acids. All contained in the nucleus of almost every cell in our bodies.
Similar efforts were undertaken for the genomes of a number of economically important organisms during this period, but cataloguing all those genes and then making sense of them proved to be entirely different endeavours. At least, until the mid-2000s, when high-throughput sequencing and the use of single nucleotide polymorphisms (SNPs) as markers became available to describe the genetic architecture of quantitative traits on a whole-genome basis. SNPs had already been recognised as useful genetic markers but with somewhat limited application because original attempts at sequencing were tedious and expensive. High-throughput methods allowed the processing of large amounts of genetic material at extremely high speeds. Some 335 million SNPs have now been identified in the human genome.
Suddenly, researchers could look at large numbers of gene markers scattered throughout all of an organism’s chromosomes. But the goal had not changed: to obtain a more accurate estimate of the genetic worth of an individual than could be developed based on previously available information (physical measurements, siblings’ performance, pedigree records, QTLs, etc.) And as the technology improves and costs come down, genome-based selection is poised to revolutionise selective breeding in aquaculture – especially in the area of pathogen resistance.
The first step in the process is usually a genome-wide association study (GWAS) or genome-wide association analysis (GWAA) – an exploratory exercise to determine which markers (typically SNPs) are associated with the trait(s) of concern. Marker information scattered across the entire genome is recorded for individuals that display a desirable trait, using high-throughput sequencing that can involve tens of thousands of markers. Once genomic profiles are generated for each individual, complex computer analyses are used to develop a relationship between individual profiles and observed performance. Ultimately, the utility of predictive estimates depends on the number of individuals and which SNPs are used when developing the genotype-phenotype relationship.
Datasets of SNPs are now available for some aquaculture species, making the task somewhat easier, and do-it-yourself molecular techniques are available to generate such datasets for other species as needed. These datasets are now being used to create SNP “arrays” that allow genome-wide profiles to be generated for large numbers of animals at the same time. An array has a large (really large) number of specific nucleic acid sequences that correspond to SNPs, each labelled with fluorescent dyes. The sequences are embedded in fixed positions on a glass surface, allowing a sample to be expressed as a colourful two-dimensional display of the SNP variants at each specific location in the genome.
Promising results
A number of recent studies illustrate the potential disease resistance improvements that can be realised with genomic selection in aquatic species. In China, researchers recently examined the genetic basis of resistance to Cryptocaryon irritans in the large yellow croaker. The parasite is a persistent disease issue for producers of this important sciaenid species, and its farming has taken on greater importance in recent years due to severe overfishing of wild stocks.
Fifteen genes with measurable impacts on resistance were identified across 12 of the species’ 24 chromosome pairs, and each explained anywhere from 1 percent to 8 percent of the overall variation observed. Resistance was associated with genes related to a variety of biological functions, including inflammatory response, apoptosis, hormone transport and bacterial resistance.
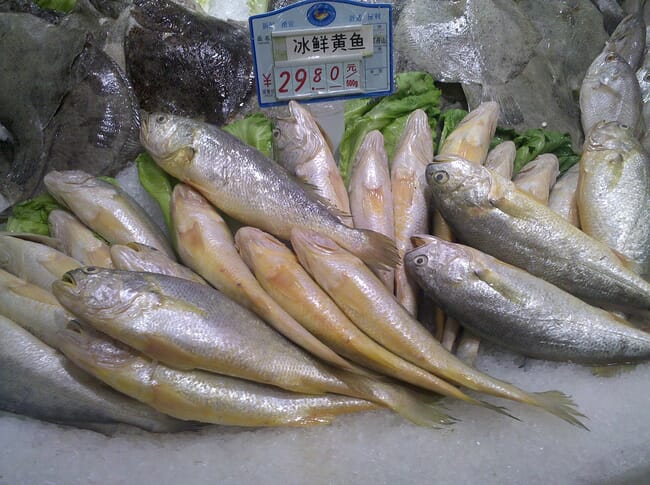
Another important aquaculture species in China is the tongue sole, (Cynoglossus semilaevis), and one of the most problematic pathogens for this species is Vibrio harveyi. A group of Chinese researchers recently reported on the potential use of genomic selection to improve resistance against this bacterium. They examined data using anywhere from 500 to 500,000 SNP markers, and determined which of four analytical methods gave the best genomic predictions of breeding values for resistance. They then developed an array that included over 38,000 SNPs. When breeding stock were genotyped using the array, the correlation between parental values and offspring performance was 0.76! Clearly, this work will allow for significant improvement of V. harveyi resistance in the tongue sole.
The architecture of genetic resistance of Atlantic salmon to infectious salmon anemia (ISA) was recently addressed by researchers from the UK and Norway. They used GWAS to produce genomic profiles of pathogen-challenged animals. While genomic selection was found to provide a higher heritability for the population in question (0.33, compared to 0.13 for pedigree-only information), the most significant gene marker explained only 3 percent of the observed genetic variance. Nonetheless, a heritability of 0.33 is more than enough to make significant progress in a selection programme.
Another recent report on genomics and disease resistance, but in a very different group of organisms, involves the potential for improvement of resistance to ostreid herpesvirus in the Pacific oyster.
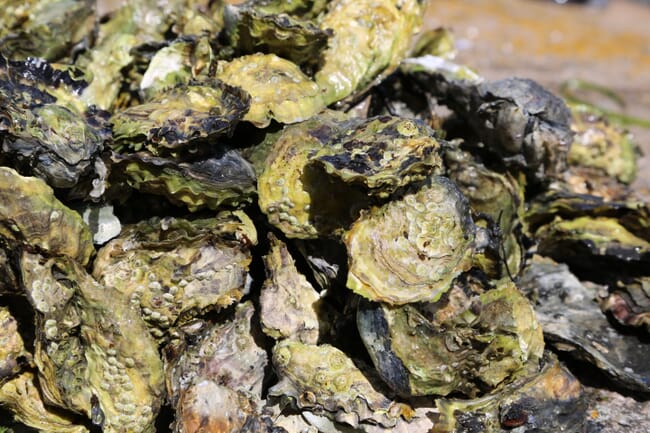
© Norden
The virus in this study is responsible for summer mortality syndrome, which causes significant economic losses on oyster farms throughout the world. Researchers from the UK and New Zealand computed genomic scores for a group of oysters and found that heritability for survival using genomic selection would be 0.37 + 0.05, compared to only 0.25 + 0.05 for selection based on pedigree information. And while initial efforts at GWAS often use many thousands of markers, this number can often be reduced, lowering costs and analytical requirements without significantly sacrificing precision.
In this study, the researchers found that reducing the number of SNP markers “had little impact on prediction accuracy”, as long as 500 or more SNPs were included in the genomic scoring analysis. The results suggest that greater improvement in resistance could be attained through genomic selection, and at a lower cost, than by using traditional pedigree and performance information.
Several months ago, researchers in China published a study examining the trade-off between cost and accuracy in genomic selection for disease-resistance in a number of aquacultured species. Since the costs of genotyping increase with the number of SNPs examined, they proposed a strategy using a subset of selected markers, based on initial GWAS results. In general, using fewer selected GWAS-identified markers actually resulted in higher prediction accuracy for offspring performance than using much larger numbers of SNPs. Of course, the optimal number of SNPs to include in a genetic profile varied from species to species and in relation to the pathogen in question, but this conclusion held true for Pacific whiteleg shrimp, Atlantic salmon and gilthead sea bream.
And speaking of shrimp, genomic selection for white spot syndrome virus (WSSV) resistance also shows great promise in L. vannamei.
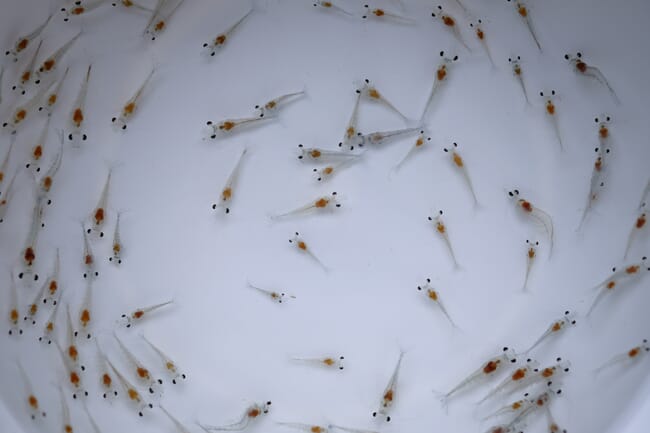
Last year, a group of researchers from Colombia, Australia and Norway examined the genetic control of WSSV resistance using a dataset of 18,643 SNPs and generated genomic scores for a number of surviving shrimp from challenge studies. Offspring of selected broodstock exhibited 51 percent survival in a challenge study, compared to 38 percent for a random population and only 25 percent for a low-resistance group of breeders.
For centuries, the great constraint in genetic improvement through selection has always been the partial disconnect between what we see and what we get. Sometimes the relationship is clear, sometimes not so much. The advent of genomic selection for aquacultured species suggests we may soon catch up to our peers in animal and plant breeding, especially with respect to disease resistance.