Introduction
The grey snapper Lutjanus griseus (L.), commonly also known as mangrove snapper, is a medium-sized snapper species which is restricted to the Western Atlantic, with its centre of distribution in the Gulf of Mexico and the Caribbean Sea ( Robins et al., 1986 and Allman and Grimes, 2002). L. griseus is a target species for commercial and recreational fisheries and the largest number of animals is being caught along the Gulf coast of Florida, with approx. 2 million lbs of grey snapper landed between 2005 and 2009 (NMFS, 2012).
Ault et al., 2002 and Ault et al., 2005 reported that the spawning potential ratio (SPR) of mangrove snapper in the Florida Keys coral-reef system was below that of a healthy stock, indicating overfishing.
Increasing mortality and decreasing size-at-age along a temperature gradient from northwest to southwest Florida has also been reported (Allman, cited by Gold et al., 2009). During fishing efforts conducted by Mote Marine Laboratory and aiming at fish population studies along the mangrove shores of the Eastern Gulf of Mexico, it was noted that juvenile mangrove snapper caught by seine nets and subsequently released, sometimes failed to recover from the stress of capture.
During a parasitological study of moribund mangrove snapper after their transport to the laboratory we detected macroscopic changes in their kidneys and large numbers of myxozoan spores in squash preparations. Myxozoans are microparasites belonging to the Cnidaria (Holland et al., 2011), and several species have been related to pathological changes and mortalities in wild and cultured fish species around the globe.
In order to better understand the influence this specific myxozoan has on its host L. griseus, we aimed to characterise this species morphologically and molecularly, determine its prevalence and intensity of infection in different age classes of fish, describe the pathological changes it causes and determine whether it can be related to mortalities.
Materials and Methods
Study Site
L. griseus were obtained within the framework of fishery-independent surveys of fish communities occupying mangrove shorelines and creeks in the Gulf of Mexico. In November and December 2012 fish were caught at the Southeast coast of the Gulf, in Florida, between Anna Maria Island in the north (27°26?36.15?N 82°41?36.09?W) and New Pass in the south (27°19?37.59?N 82°35?14.06?W). Fishing was conducted in shallow areas between the keys and the mainland, and in the canals connecting this area with the Gulf. The capture areas were characterised by sandy or muddy sediment, sometimes covered with sea grass, and a depth of 1–10 m. Seine nets were used and some fish were caught by line fishing from the shore. A total of 27 L. griseus were captured for parasitological study. On thin sections of otoliths, annual growth zones (annuli) were examined and the fish were ascribed to three year-based age classes: age-0 (n = 12; total length (TL) 7.5–10.5 cm), age-1 (n = 10; TL 20.6–25.2 cm) and age-2 (n = 5; TL 23.2–34.0 cm). Thereby, the relation between age and TL was in accordance with the results from other studies ( Burton, 2001, Fischer et al., 2005 and Jones et al., 2010).
Parasitological Study – Sampling Procedures and Morphological Details
Fish were transported live to the aquaculture facilities of Mote Aquaculture Research Park (MAP) in large, aerated containers and were transferred to an isolated holding tank on site. All specimens were maintained in the facilities for a maximum of 48 h before they were euthanised by an overdosis of MS222. Thereafter, blood was taken from the caudal vein, using a sterile syringe, and 4 ?l of blood were collected in 100% ethanol. Fish were weighed, measured and necropsied. Fresh kidney smears were observed under the light microscope at ×400 to ×1000 magnification. Spores were measured on digital images using ImageJ v.1·44p (Wayne Rasband, http://imagej.nih.gov/ij) calibrated against a digital image of a graticule. Measurements (n = 15 spores per fish, 3 L. griseus) were obtained following the guidelines of Arthur and Lom (1989) and Sitjà-Bobadilla and Álvarez-Pellitero (1994). Kidney tissue from one heavily infeal times. Finally, a pellet consisting predominantly of spores was collected and left to settle for 30 min onto poly-l-lysine coated slides and coverslips. Some sporcted fish was minced up in an Eppendorf tube using a peston and incubated with sterile sea water in order to disrupt the tissues and release spores from it. After 3 days the solution was pelleted at 2000 g, the supernatant was discarded and the pellet washed severe preparations were fixed in methanol and incubated with DAPI overnight to be able to count the number of sporoplasms in the spores. Lysine-coated coverslips with spores were fixed in 2.5% glutaraldehyde and processed for scanning electron microscopy (SEM) as described in Alama-Bermejo et al. (2009). For molecular analyses, blood obtained from the caudal vein and small kidney pieces of each fish were fixed in 100% ethanol. Furthermore, kidney pieces of all age-0 fish were fixed in 10% neutral buffered formalin for histological analyses.
Molecular Analyses and Phylogeny of Parasite Isolates
Ethanol–fixed blood was pelleted and the ethanol left to evaporate before adding TNES urea buffer (Asahida et al., 1996) to the tubes. Ethanol-fixed kidneys were briefly dried on filter paper and transferred to TNES urea buffer. All samples were stored in buffer for several days, until DNA extraction. DNA was extracted using a simplified phenol-chloroform extraction protocol (Holzer et al., 2004) and, after drying, DNA was redissolved in RNAse/DNAse-free water. Myxozoan small subunit ribosomal DNA (SSU rDNA) was amplified in three overlapping segments using universal and semi-specific primers designed in previous studies. Primers ERIB1 (5?-ACCTGGTTGATCCTGCCAG-3?) and ERIB10 (5?-CTTCCGCAGGTTCACCTACGG-3?) (both Barta et al., 1997) were used in the first round PCR at 62 °C annealing temperature (AT). This PCR product was used as a template in three different nested PCR reactions: (1) MyxGP2F (5?-TGGATAACCGTGGGAAA-3? Kent et al., 1998) and Act1R (5?-AATTTCACCTCTCGCTGCCA-3? Hallett et al., 2002) at 58 °C AT, (2) ERIB1 and BasalSphCladeSSU1850R (5?-AACCRATACCCRTACAYRGRRRGTGC-3? Bartošová et al., in press) at 53 °C AT, and (3) Myxgen4F (5?-GTGCCTTGAATAAATCAGAG-3? Diamant et al., 2004) and ERIB10 at 60 °C AT. PCRs were conducted in 20 ?l reactions with 0.025U ?l?1 Titanium Taq DNA polymerase and 10× buffer which contained 1.5 mM MgCl2 (BD Biosciences Clontech), with 0.2 mM of each dNTP, 0.5 mM of each primer, and 50–150 ng of template DNA. Denaturation of DNA (95 °C for 2 min) was followed by 30 cycles of amplification (95 °C for 40 s, AT (see above) for 40 s, and 68 °C for 2 min) and terminated by a 4 min extension (68 °C). Overlapping fragments of the kidney myxozoan of three L. griseus individuals were sequenced in the sequencing facility of the Faculty of Science at the Biological Centre of the Academy of Sciences of the Czech Republic. The three partial SSU fragments were self-assembled and aligned in SeqMan II (DNASTAR, Inc.). The obtained consensus sequence was submitted to the BLAST tool on GenBank™ to check for the closest relatives. Based on this, the sequence was aligned to a subset of SSU rDNA sequences from a previous alignment (Bartošová et al., in press) which were used as a profile in Clustal X version 1.18 (Thompson et al., 1997). The alignment was manually edited and the ambiguous regions were excluded in BioEdit (v7.0.5.2; Hall, 1999). To estimate phylogenetic relationships to other myxozoans, we performed Maximum Parsimony (MP) analysis in PAUP* (v4.b10; Swofford, 2001), using a heuristic search with random taxa addition, the ACCTRAN option, TBR swapping algorithm, all characters treated as unordered and gaps treated as missing data. The Maximum Likelihood (ML) analysis was calculated in RAxML (Stamatakis, 2006) using GTR + ? model. Clade support values were calculated from 1000 bootstrap replicates with random sequence additions in both MP and ML analyses. The Bayesian Inference (BI) analysis was performed in MrBayes (v. 3.0; Ronquist and Huelsenbeck, 2003) using the GTR + ? + I model of evolution. Posterior probabilities were estimated from 1,000,000 trees via two independent runs of four simultaneous Markov Chain Monte Carlo chains with every 100th tree saved. The burn-in period (50,000 generations) was determined in Tracer (v. 1.4.1; Rambaut and Drummond, 2007).
Based on differences in the variable regions of the SSU rDNA gene region, specific primers were designed for the polysporoplasmid parasite from L. griseus. SmoteF (5?-TCGTGTGGCTAGACTGGCATC-3?) and SmoteR (5?-AGTGAGAGAGGGAGAAGGCAC-3?) were then applied in PCR to determine whether the parasite occurred in the blood and whether the prevalence of infection observed by microscopy was the same as determined by PCR. PCR conditions were as above with 66 °C AT and a shorter elongation step (40 s). To test the specificity of the newly designed PCR primers, DNA extractions of twelve species belonging to Sphaerospora sensu stricto were checked for cross-reactivity. These included Sphaerospora sparis (Sitjà-Bobadilla and Álvarez-Pellitero, 1995), Sphaerospora elegans Thélohan (1892), Sphaerospora hankai Lom et al. (1989), Sphaerospora molnari Lom et al. (1983), Sphaerospora angulata Fujita (1912), Sphaerospora dykovae (Lom and Dyková, 1982) and four unidentified/undescribed Sphaerospora spp. (GenBank accession numbers JX286621-22, JX286625-26).
Intensity of Infection and Pathological Changes
On fish dissection, the macroscopic appearance of the kidney was reported for each individual. Formalin-fixed tissues were dehydrated through an ethanol series, transferred into xylene and embedded in paraffin. Five ?m sections were cut and stained with Haematoxylin and Eosin (H&E) and according to Giemsa. Stained tissue sections were mounted in Pertex and scrutinised for histopathological changes. The intensity of infection was evaluated semi-quantitatively according to a scale ranging from 1 to 3. The scale was based on the number of parasite stages per Bowman’s capsule in each infected fish of the age-0 age class.
Results
Parasite Characterisation
Spores of the myxozoan parasite detected in the kidney of L. griseus matched the recently revised generic description of Sphaerospora which includes the synonymized genus Polysporoplasma (Bartošová et al., in press), however, spore size and host did not match previous descriptions of polysporoplasmic Sphaerospora spp. (all described in the Mediterranean and the eastern Atlantic).
3.1.1. Sphaerospora motemarini n. sp.
3.1.1.1. Description
Spores (Fig. 1A–C) slightly thicker and wider than long, two subspherical polar capsules in a plane which lies perpendicular to the plane of the suture, polar filament in 5–6 turns. Spore valves thick with ornamentation posterio-laterally on each valve (Fig. 1A and C). Ornamentation in the shape of pits providing an opening to a multilayered canal system (Fig. 1D). Apical part of valves thickened at suture line (Fig. 1A), posterior part of spore with two prominent bulges (Fig. 1A), indicating the location of the valvular nuclei which persisted also in mature spores. Consistent number of six mononuclear sporoplasms restricted to the central part of the spore (Fig. 1B). A line drawing summarizing all spore characteristics is provided in Fig. 2. Spore measurements in ?m, providing mean and range (in parenthesis): Spore length 16.31 (13.85–18.37), spore width 19.85 (18.23–21.65), spore thickness 20.41 (18.83–22.03), polar capsule length 6.47 (5.76–7.41), polar capsule width 6.43 (5.55–7.54). Spores developing in spherical to oval disporous pseudoplasmodia (Fig. 1C) measuring 41.12 (36.45–45.79) when containing mature or semi-mature spores. Sporoblasts inside pseudoplasmodium croissant-shaped (Fig. 1C) with a fine connection between the posterior median point of spore and sporoblast envelope. Earliest stages of pseudoplasmodia (Fig. 1E) lacked spores but contained several refractile granules and were smaller than those containing spores, with a diameter of 19.79 (15.94–27.54).
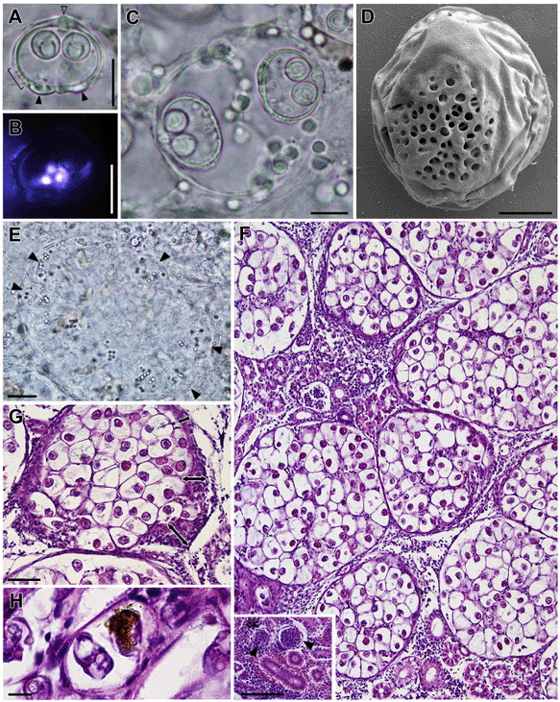
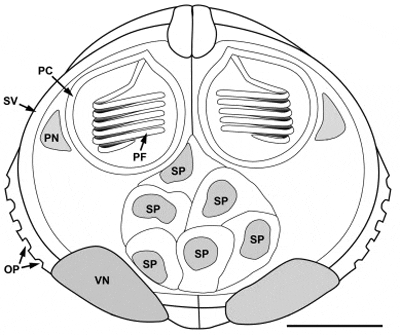
Location
Spore-forming pseudoplasmodia were located predominantely in the capillaries of the glomerular tuft of the renal corpuscles, rarely in the renal tubules or the interstitial tissue of the kidney. Invasive stages of unknown morphology in the blood (PCR). The PCR assay designed for S. motemarini n. sp. is highly specific and did not produce PCR amplicons in any other Sphaerospora sensu stricto sp. tested for cross-reactivity.
Type host
Grey snapper or mangrove snapper Lutjanus griseus (L.); S. motemarini n. sp. was not detected in other fish species (n = 14) screened parasitologically during the survey of fish communities occupying mangrove shorelines, however, these did not contain other members of the Lutjanidae.
Locality
West coast of Florida, Gulf of Mexico.
Etymology
In honor of MOTE Marine Laboratory (Florida, Sarasota) who provided substantial support for the present study.
Phylogenetic relationships
The SSU rDNA sequence obtained from S. motemarini n. sp. showed large inserts in the variable regions of the SSU rDNA, resulting in a total length of 3090 bp. Phylogenetic analyses of S. motemarini n. sp. showed that this species is a member of the Sphaerospora sensu stricto clade of myxozoans as defined by Jirk? et al. (2007) and Bartošová et al. (in press). Thereby, S. motemarini n. sp. is most closely related to Sphaerospora (syn. Polysporoplasma) sparis (Sitjà-Bobadilla and Álvarez-Pellitero, 1995) from Mediterranean gilthead seabream Sparus aurata L. and forms a well supported monophyletic clade with all other polysporoplasmid Sphaerospora spp. sequenced to date (Fig. 3).
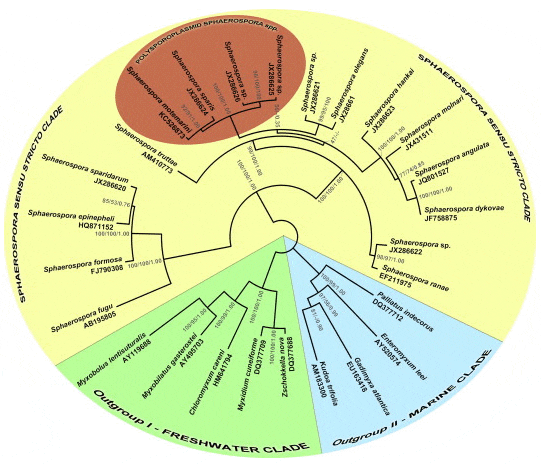
Material deposited
Two microscopic slides with sections of 24 h formalin-fixed kidneys with heavy S. motemarini n. sp. infection, stained with Giemsa (1) and H&E (1), 100% ethanol fixed kidney with heavy infection with S. motemarini n. sp. for DNA analysis, includes synbiotype. Protistological Collection of the Institute of Parasitology, Biology Centre of the Academy of Sciences of the Czech Republic: specific reference number IP ProtColl P21. SSU rDNA sequence deposited on GenBank™ (Acc. Nr. KC526873).
Parasite prevalence and intensity per age class
Prevalence was determined as 83% (10/12) in the age-0 class, 40% (4/10) in the age-1 class and 0% (0/5) in the age-2 fish. Thereby, visual examination and PCR obtained the same result. Blood samples only tested positive for S. motemarini n. sp. in the age-0 class, with a prevalence of 25% (3/12) determined by PCR. Table 1 shows the severity of S. motemarini n. sp. infection in the youngest fish, following a scale from 1 to 3 which relates to a determined number of pseudoplasmodia in 10 Bowman’s capsules per tissue section (see Table 1). Four of the 12 fish had a serious degree (3) of infection with up to 132 pseudoplasmodia in their strongly enlarged renal corpuscles.
Table 1. Intensity of Infection of Age-0 Grey Snapper and Stress-Induced Mortalities
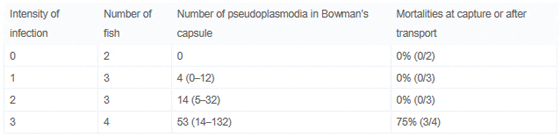
Pathology
Twenty five percent (3/12) of the smallest fish (age-0) died overnight in the MAP aquaculture facilities. These fish exhibited macroscopic changes of the kidney, which manifested themselves in form of enlarged, pale kidneys demonstrating a granular surface and consistency and exhibiting massive numbers of spores (intensity of infection scale 3, see Table 1) as well as considerable histopathological changes. Macroscopic changes were not detected in age-1 and age-2 snapper. Depending on the number of parasites and the stage of parasite development in age-0 fish, we observed different degrees of histopathology. H&E stained tissue sections showed gradual hypotrophy and destruction of renal corpuscles which was clearly linked to spore maturation. With increasing number of pseudoplasmodia and with maturation of spores the Bowman’s capsule was thickened and mesangial cells were found to proliferate (Fig. 1G). In the final stage of disease, the renal corpuscles were massively enlarged, increasing their diameter 2–11 fold from their normal condition (Fig. 1F). At this point, capillaries were devoid of any blood cells and the Bowman’s space was filled with masses of pseudoplasmodia and spores (Fig. 1F–G). Inflammatory host tissue reactions included leucocyte infiltration involving predominantly eosinophilic granulocytes and melanomacrophages. The latter were found to phagocytize individual spores (Fig. 1H). The pathological changes observed were linked directly to the presence of parasites and thus prompt us to state that S. motemarini n. sp. causes severe glomerulonephritis in L. griseus.
Discussion
The present study clearly demonstrated that the newly described myxozoan species S. motemarini n. sp. causes serious glomerulonephritis in the kidney of grey snapper, L. griseus. We were also able to relate heavy infections, which possibly interfere with a healthy kidney function, to mortalities in stress situations. Thereby, the pathology related to S. motemarini n. sp. infection in L. griseus was similar to that described for Sparus aurata infected with S. sparis (Palenzuela et al., 1999), its closest relative. However, in the present study we showed that predominantly juvenile fish (i.e. age-0) were affected. The absence of invasive blood stages in age-1 and age-2 fish suggests that older fish become immunized to S. motemarini n. sp., a feature occurring in several Sphaerospora sensu stricto spp. (e.g., Odening et al., 1988 and McGeorge et al., 1996). Alternatively, host habitat changes may result in age-1 and age-2 grey snapper receiving a lower dose of infective actinosporean stages, as they move away from the mangroves. Juvenile grey snapper remain in estuarine nursery areas, such as seagrass beds or mangrove shorelines (e.g. Whaley et al., 2007 and Faunce and Serafy, 2007), while subadults move into channels and eventually migrate to offshore structured habitats, where they reach maturity ( Manooch and Matheson, 1981 and Domeier and Colin, 1997).
Typically, polysporoplasmid Sphaerospora spp. develop in the glomeruli ( Sitjà-Bobadilla and Álvarez-Pellitero, 1995 and Palenzuela et al., 1999) while other sphaerosporids most frequently inhabit the renal tubules ( Sitjà-Bobadilla and Álvarez-Pellitero, 1994 and Lom and Dyková, 2006). The earliest plasmodial stages detected in the glomeruli of L. griseus were of small, uniform size and we assume they had recently entered the glomeruli from the general vascular system. While proliferative blood stages are common in sphaerosporids ( Baska and Molnár, 1988, Supamattaya et al., 1993, McGeorge et al., 1996 and Eszterbauer et al., in press) they have not yet been described from the polysporoplasmid spp. of the genus. We hereby proved by PCR that S. motemarini n. sp. occurs in the blood, however, it is unclear whether it uses the blood stream for transport to the target organ or if pre-sporogonic development takes place. Spore formation was observed in the renal corpuscles and surprisingly, spores were only occasionally detected in the tubular lumen. This poses the question whether S. motemarini n. sp. spores are actually released via the urinary system or whether this myxozoan is dependent on death or predation of the host for transmission to the next host. The neck segment which connects the renal corpuscule with the proximal tubule is relatively narrow and spores of all known polysporoplasmid Sphaerospora spp. are quite large (minimum diameter 16–21 ?m; Sitjà-Bobadilla and Álvarez-Pellitero, 1995; present study) which may prevent the release of the spores from the renal corpuscules. The fact that all polysporoplasmid spores have extremely thick and resistant valves suggest that they may be designed to resist the digestive enzymes of a predator.
We clearly demonstrated that heavy infections of L. griseus with S. motemarini n. sp. cause pathological effects, and in combination with stress (capture, netting), can cause mortality amongst young-of-year. The compromised condition of age-0 grey snapper suffering from severe glomerulonephritis may also make them an easier target for predators.
It is noteworthy that grey snapper, as many other exploited reef fishes, is especially susceptible to the effects of overfishing due to unique life-history characteristics such as depth and habitat preferences, longevity, and a high probability of mortality for undersized individuals (Coleman et al., 1999 and Bartholomew and Bohnsack, 2005). Overfishing seems to occur in the south of Florida (Ault et al., 2002 and Ault et al., 2005), and fishing mortality in south Florida has been found to be much higher than fishing mortality in northeast Florida (Manooch and Matheson, 1981 and Burton, 2001). While there is evidence that grey snapper in south Florida may be depleted, overall abundance levels for the Florida Atlantic coast and the Gulf coast populations remain uncertain since there are no estimates of biomass in relation to target biomass reference points. Only one publication mentions an increasing abundance of grey snapper over the past 30 years (Tolan and Fisher, 2009). This study was conducted in the north-western Gulf of Mexico and adjacent estuaries and related the population growth to increased winter seawater temperatures (Tolan and Fisher, 2009). Winter temperatures in this area are generally around 10 °C colder than at our sampling sites on the central eastern Gulf coast (NOAA temperature data). Environmental temperature is a critical factor that affects the function and efficiency of biological and physiological processes of poikilothermic animals, including disease resistance. At the same time, proliferation rates of pathogens are greatly influenced by temperature. It has been shown that increased temperatures allow myxozoans to proliferate more rapidly in both, their vertebrate and invertebrate hosts (Ferguson, 1981, Redondo et al., 2002, Yasuda et al., 2002, Foott and Hedrick, 1987, Tops et al., 2006 and Ray et al., 2012). Further study is warranted to quantitatively correlate S. motemarini n. sp. infestation rates in grey snapper found in different habitats, and understand how stressful events such as high temperatures, fishing, and ontogenetic migrations may influence population dynamics of host and parasite.
July 2013