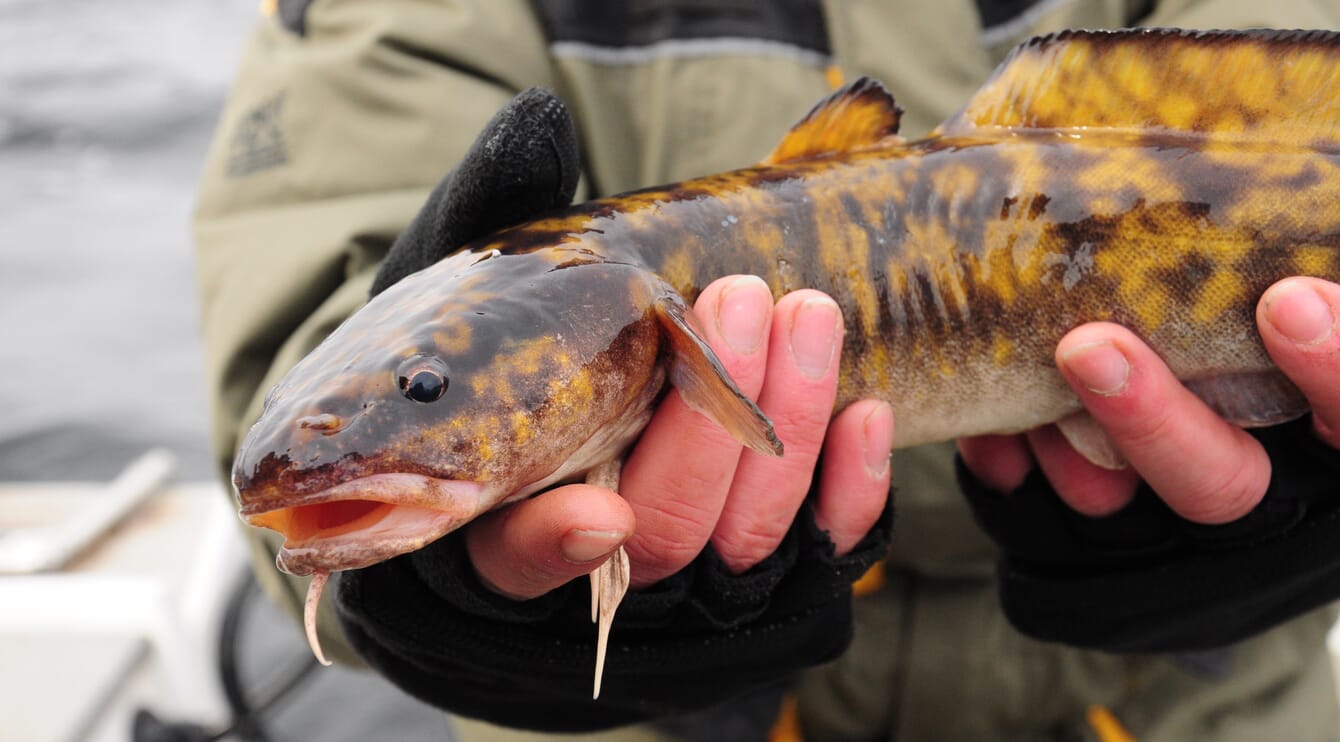
There are two major sub-species of this freshwater cod-like fish, one ranging from Europe eastward to western Canada (L. lota lota), and another in North America at somewhat lower latitudes (L. lota maculosa). © Shutterstock
The burbot, Lota lota, has a circumpolar distribution, occurring naturally in rivers and lakes across the northerly regions of Europe, Asia and North America. The phylogenetic relationships of the species are still somewhat unclear, but there appear to be two major sub-species, one ranging from Europe eastward to western Canada (L. lota lota), and another in North America at somewhat lower latitudes (L. lota maculosa). Of all the cod-like fishes, it is the only species that has adopted a freshwater life history – a transition that took place somewhere in Europe between 5 and 10 million years ago. Although the species has thrived in freshwaters, it retains many of the life history characteristics of its marine relatives, such as high fecundity (100,000 to 800,000 eggs per kg female body weight, with the largest females producing up to 3 million eggs), pelagic larval stages and a need for very cold spawning temperatures.
The burbot has long been a highly prized fish in Europe and elsewhere, but it is now found only in exclusive niche markets and the kitchens of dedicated sport fishermen and -women. Burbot flesh is similar to that of cod and haddock in texture, but with a sweeter flavour reminiscent of lobster, and its liver and roe are considered delicacies in culinary circles.
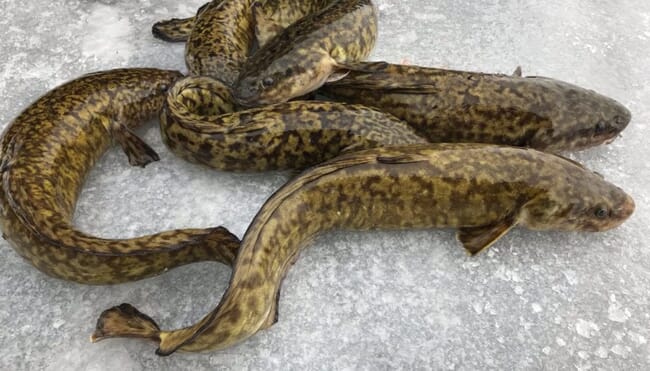
Burbot has a circumpolar distribution, occurring naturally in rivers and lakes across the northerly regions of Europe, Asia and North America
Over the past century, burbot populations have experienced steep declines in both Europe and North America – due primarily to fishing pressure, habitat degradation (especially impoundments), pollution and impacts from invasive species. Burbot spawn naturally at temperatures below 2oC, typically once every year, and they are generally restricted to the watersheds in which they have hatched. As a result, climate change trends will be particularly problematic for many wild populations of this psychrophilic species.
Captive spawning
Research aimed at restoring and reestablishing populations has progressed in North America and Europe over several decades, allowing for the development of hatchery protocols to provide fingerlings for conservation efforts. As has been the case with many other aquatic species, interest in adapting these techniques for commercial aquaculture has emerged, with ongoing research in places as diverse as China, Poland, Hungary, Germany, Switzerland, Colorado and Idaho. Commercial hatcheries producing burbot fry are operating in Belgium (AquaLota) and Germany (LotAqua), and a new facility is starting up near Vancouver, Washington (Evergreen Aquatics).
In the US, the University of Idaho has taken a lead role in burbot research, mainly focusing on understanding burbot at a commercial aquaculture level © Luke Oliver
Some of the earliest captive spawning investigations with burbot were conducted in Czechoslovakia in the 1980s. Results were published in regional journals in Czech and Slovak. Many practices and equipment developed previously for other species are applicable to burbot. Kucharczyk et al. (2018) evaluated spontaneous and hormone-induced spawning of wild-caught burbot broodstock and found that application of salmon gonadotropin releasing hormone analogue (sGnRHa) resulted in 100 percent ovulation, but volitional spawning also occurred in 55 percent to 65 percent of female fish that were not injected. Non-ovulating fish given the opportunity to spawn volitionally were subsequently injected and induced to spawn at rates of 43 percent to 57 percent. The highest embryo survival rates in these trials (up to 84 percent) were obtained from females that were hormonally induced without the opportunity for volitional spawning.
Similarly, Kucharczyk et al. (2022) presented an account of artificial reproduction in both wild and cultured broodstock from a Polish population of burbot. After captive maturation using photothermal manipulation and a hormone treatment, fertilisation rates were over 95 percent for both groups. Survival to eyed egg stage and subsequent hatchability was approximately 92 percent and 91 percent, respectively. Oliver et al. (2021) reported no impacts on egg quality or larval survival when the spawning cycle of captive burbot was shifted by six months through photoperiod and temperature control.
Although the species has thrived in freshwaters, burbot retains many of the life history characteristics of its marine relatives, including high fecundity with females producing 100,000 to 800,000 eggs per kg body weight, with the largest females producing up to 3 million eggs © Luke Oliver
Larval culture
Burbot eggs are slightly sticky, which must be accommodated for prior to incubation. Burbot require a long incubation period (6 to 8 weeks) and an extended larval culture phase due to 1) the cold temperatures needed by the species and 2) the fact that larvae are extremely small (0.4 mm) at hatching.
After hatching, temperature is raised to 10oC for larval and juvenile culture. Larvae are typically fed with brine shrimp nauplii until weaning begins at 35 to 60 days post-hatch, but choice of feeds is important because some artificial diets are completely rejected (Wocher et al. 2011). Some research suggests CO2 must remain below 20 and constant light is beneficial until fish are weaned. Early research on larval culture found that feeding only Chlorella sp. for the first few days, followed by rotifers and Artemia, provided the highest survival after 35 days post-hatch (Harzevili et al. 2003). More recently, Jensen et al. (2011) reported satisfactory results when offering several commercially available larval diets, when compared with live Daphnia.
These and other authors suggest that grading must begin very early in the hatchery, in the larval culture phase actually, inasmuch as high levels of cannibalism (>50 percent) are not uncommon for burbot during the first few weeks. Barron et al. (2013) confirmed the importance of grading during the initial larviculture period. Although survival of ungraded larvae in their control group averaged 59.3 percent, survival of graded larvae averaged 74.3 percent and 93.3 percent for larvae passing through or retained by graders, respectively. However, grading was less effective for metamorphosing juveniles due to the presence of several extremely large individual fish, suggesting the need for additional segregation of the very largest juveniles.
Research at the University of Idaho has found that burbot larvae require live feed – rotifers and Artemia – for the first 30-50 days post-hatch © Luke Oliver
Grow-out
A water temperature of 15oC appears to be ideal for grow-out. Once completely weaned to artificial diets, which may take up to 100 days depending on various conditions, cannibalism is minimal if proper feeding and grading practices are used. Juvenile burbot exhibit acceptable growth at dissolved oxygen levels of 5.0 ppm, but levels of 7.0 ppm or higher are recommended for grow-out (Vaage and Myrick 2022), and levels below 2.0 ppm cannot be tolerated for more than a few hours. Perhaps even more importantly, juvenile burbot can acclimate slightly to low levels of unionised ammonia, but this form of ammonia must be maintained below 0.03 ppm to ensure acceptable feed intake and growth (Vaage and Myrick 2021).
There are many research-based and anecdotal reports of burbot exhibiting feed conversions of less than 1:1. In a recirculating nursery system, the best growth and feed conversion results appeared to be achieved when feeding 2.0 percent body weight daily (Tejchel et al. 2014). Although juvenile burbot may have a higher requirement for marine proteins, sub-adults appear to perform well on commercial trout diets (Bruce et al. 2020). Research into burbots’ nutritional requirements is ongoing, but initial studies indicate that 25 percent substitution of fishmeal with soybean meal or soy protein concentrate does not impact growth, intestinal integrity or immune function (Bruce et al. 2021).
Although earlier life stages can be maintained at up to 50 kg per m3 and some researchers have reported success at up to 100 kg per m3, burbot adopt a bottom-dwelling lifestyle as they increase in size. At that point raising them is more like flounder culture, in that they use all the available space on the bottom of the tank or raceway. If water depth is sufficient to accommodate additional, elevated flat surfaces, more fish can be produced with the same floor space, as long as suitable water quality can be maintained. Acceptable growth has been reported when stocking at up to 18 kg per square metre (Wocher et al. 2011).
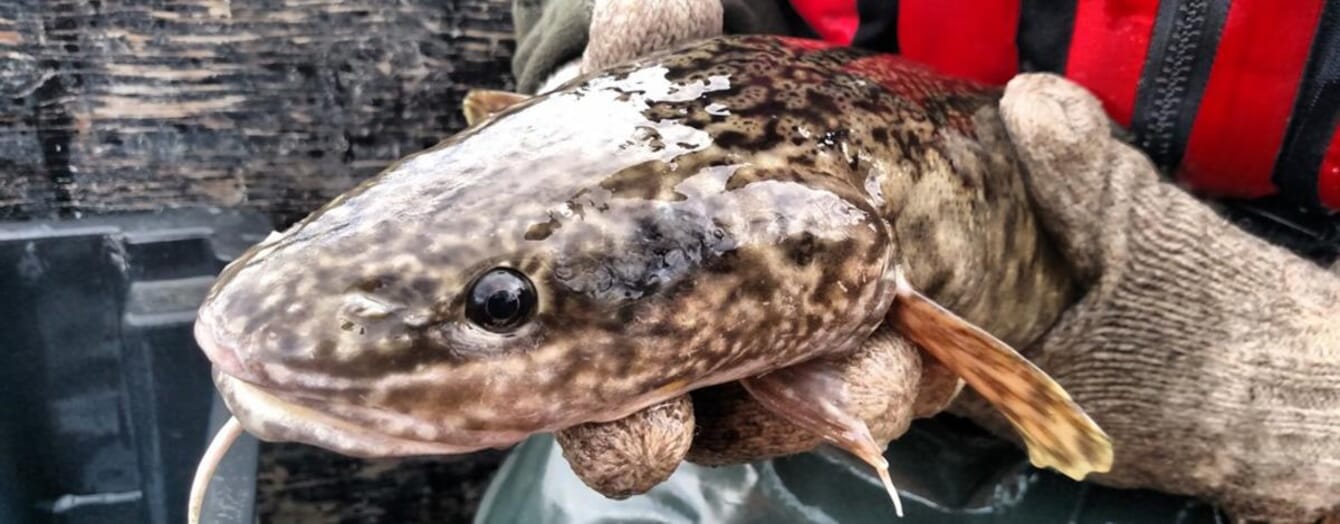
© Idaho Fish and Game
Aeromonas infections are occasionally problematic during grow-out (Terrazas et al. 2018), but burbot also seem to be resistant carriers of Flavobacterium columnare (Bruce et al. 2020). These studies confirmed previous results reported by Polinski et al. (2010), who found that burbot could also harbour infectious haematopoietic necrosis virus (IHNV), infectious pancreatic necrosis virus (IPNV) and Renibacterium salmoninarum. This could complicate biosecurity if attempting to culture them in the same facilities as trout or other salmonids. Some disease issues have also been reported from RAS culture (Pietsch et al. 2020).
Looking forward
As genomic characterisation and selection methods advance, new possibilities for domestication and conservation of aquatic species continue to emerge, and burbot is no exception to this technological trend. Han et al. (2021) reported on the first chromosome-level genome assembly for the fish, with a focus on those genes involved in adaptation to a freshwater life history. Gao et al. (2021) applied comparative transcriptomic analysis to examine temperature acclimation in a population of burbot from the Irtysh River Fish Breeding Facility in China. They identified heat shock protein HSP70 as an important indicator of temperature acclimation capability, and found that heat stress upregulated immune-related gene expression.
In the US, the University of Idaho has taken a lead role in burbot research. Dr Kenneth Cain, distinguished professor in the Department of Fish and Wildlife Sciences, has been involved in most of these activities, and former student Dr Luke Oliver has remained with the programme as a post-doc. Although earlier work focused on techniques required for population restoration, the University's current burbot research efforts have shifted somewhat, according to Dr Oliver.
“Overall, our research is focused on understanding burbot at a commercial aquaculture level. Specifically we have recently performed experiments that increase our understanding of nutritional requirements, reproductive control, early-life culture, and immunological parameters. Our current research is investigating tetraploid induction, immersion vaccination to Aeromonas species, and elucidating issues with swim bladder over-inflation,” Oliver explains.
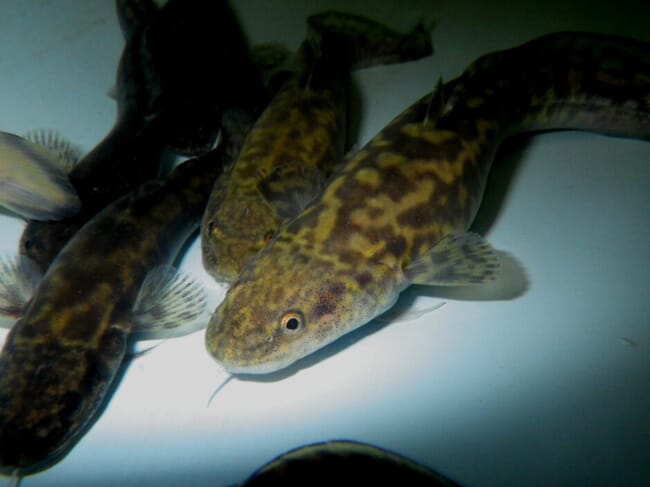
Research by Vaage and Myrick (2021) has found that juvenile burbot can acclimate slightly to low levels of unionised ammonia, but this form of ammonia must be maintained below 0.03 ppm to ensure acceptable feed intake and growth © USFWS Mountain Prairie
“At this time, burbot larvae require live feed – rotifers and Artemia – for the first 30-50 days post-hatch. This presents a large investment of time, money and skill. Recently, we have observed that rotifers or Artemia, but not both, can be replaced with a commercial diet. However, a significant reduction in survival accompanies this.
“Additionally, burbot have yet to be domesticated for commercial production and show a modest amount of diversity in growth rates. We have observed individual burbot that reach market size in 18 months; however, these individuals are the exception. A selective breeding programme may be necessary, as a high growth rate potential exists in the species, to see production rates approaching those observed in commercial trout production.”
When asked about previous or current collaborators, Oliver notes: “We collaborated with a team from Switzerland – Christian Zbinden and Patrick Kull of VierFisch Gmbh. They shadowed us in our laboratories as we demonstrated our spawning techniques and instructed them on considerations for general culture of the various life stages. I have continued to provide long-distance assistance to Patrick as he has built his company. We are open to collaborations, domestic and abroad, to get burbot to the main stage of commercial aquaculture.
“Domestically, we are collaborating with Colby Johnson of Evergreen Aquatics through an SBIR grant. Colby is currently finishing construction on a burbot broodstock and fingerling production facility and will soon begin research into larval swim bladder over-inflation,” he adds.
Dr Cain summed things up like this: “Burbot represent a unique new freshwater aquaculture species with high market potential that can be reared in a variety of culture systems designed for cold or cool water species.”