Low oxygen zones are ubiquitous features in coastal and open ocean environments. Within coastal zones, the excessive delivery of nutrients from agriculture and urban centers stimulates algal productivity, and the subsequent microbial degradation of this organic matter reduces oxygen levels, contributing towards hypoxia. Hypoxic regions of coastal zones have expanded in recent decades to the detriment of resident organisms and fisheries. In the open ocean, oxygen minimum zones are persistent features of subsurface waters with rates of microbial oxygen consumption that exceed oxygen ventilation rates and are expanding due to ocean warming. A less frequently considered consequence of microbial respiration is the production of CO2 and the resultant reduction in pH through the formation and dissociation of carbonic acid. Through the process of respiration, levels of CO2 and O2 are stoichiometrically linked in marine ecosystems, being subsequently altered by differences in gas exchange and chemical equilibria. Recent studies have indeed shown a close correspondence between low oxygen and acidification in many coastal and open ocean systems, with pCO2 levels in low oxygen zones often of a magnitude greater than predicted for surface oceans later this century (>1,000 μatm). The continued combustion of fossil fuels and subsequent warming of the planet are expected to expand low oxygen and acidified regions in the world’s oceans this century.
Despite the common co-occurrence of hypoxia and acidification in marine systems, their concurrent effects on ocean life are poorly understood. Acidification negatively impacts an array of marine organisms. Oxygen is required to sustain aerobic life, and the thresholds at which low dissolved oxygen causes mortality and/or harm have been established for many marine organisms. However, the large majority of laboratory studies assessing the effects of low oxygen on marine organisms have manipulated oxygen levels by administering nitrogen gas, a process that reduces both oxygen and pCO2 levels and thus significantly increases, rather than decreases seawater pH (Figure 1). Hence, the majority of hypoxia studies performed to date have created a laboratory condition (low oxygen, low CO2, high pH) that does not reflect the actual physiological challenges to marine life in low oxygen zones (Figure 1).
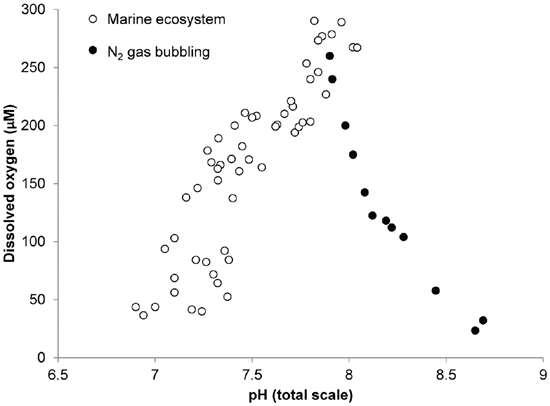
Here, we examine the concurrent impacts of acidification and low oxygen on the early life stages of two calcifying bivalves: bay scallops, Argopecten irradians, and hard clams, Mercenaria mercenaria. As foundational species within coastal ecosystems, calcifying bivalves such as those studied here are of substantial value to man: mollusk harvests approach one billion USD annually in the US alone with ecosystem services far exceeding that value. The negative impacts of climate change on the early life history stages such as those studied here may promote population bottlenecks in bivalve populations. While early life stage clams and scallops are sensitive to ocean acidification, the effects of low oxygen on these organisms under acidified conditions have yet to be examined. For this study, we specifically hypothesized that early life growth, development (metamorphosis), and survival of these bivalves would be differently affected by hypoxic vs. acidified conditions, and that concurrent hypoxia and acidification would have additive negative effects. During experiments, levels of dissolved oxygen, pCO2, and pHT (pH measured on the total scale) in seawater were achieved by mixing air, CO2, and N2 gases, as well as by amending naturally hypoxic and acidified coastal water from the Forge River Estuary, NY, USA, with aeration and sodium carbonate (Figure 2; Tables S1, S2, S3, S4).
Results
In experiments where air, CO2, and N2 gases were mixed to create hypoxic and acidified conditions for A. irradians larvae, levels of dissolved oxygen were significantly lower in the hypoxic and combined low oxygen and acidified treatment (36.5±8.93 μM) relative to the other treatments (260±23.5 μM), while levels of pH were significantly lower in the acidified and combined low oxygen and acidified treatments (pHT= 7.53±0.04) relative to the other treatments (8.00±0.03; p<0.05; Figure 2A). These lower pH levels were accompanied by aragonite saturation states (0.79±0.06) that were significantly lower than the control and low oxygen treatments (1.94±0.14; p<0.05; Table S1). Under these conditions, larval scallops (A. irradians) experienced significantly reduced survival (Figure 3A; p<0.05; Table S5) in response to acidification but not hypoxia (Figure 2A), and there was no interaction between the treatments (Table S5). Specifically, daily mortality rates of individuals exposed to normal pH and oxygen, low oxygen, low pH, and low oxygen combined with low pH treatments were 1.5, 1.5, 1.9, and 1.8 per cent per day while total survivorship after 40 d was 42 per cent, 41 per cent, 24 per cent, and 20 per cent, respectively (Figure 3A). In contrast to survival, low oxygen levels significantly reduced the size and delayed metamorphosis of scallop larvae (Figures 3B, 4A; p<0.001 for each, respectively Tables S6, S7), while low pH alone did not. The treatments interacted to yield less than additive percentage of metamorphosed larvae while there was no interaction with regard to size (Tables S6, S7). After 25 d, 72 per cent and 56 per cent of surviving individuals had metamorphosed in the control and acidified treatments, while none had metamorphosed in the low oxygen treatments with or without low pH (Figure 3B). After 40 d, 97 per cent and 91 per cent of individuals had metamorphosed in the control and acidified treatments, while only 9 per cent and 2 per cent had done so in the low oxygen treatments with or without low pH (Figure 3B).
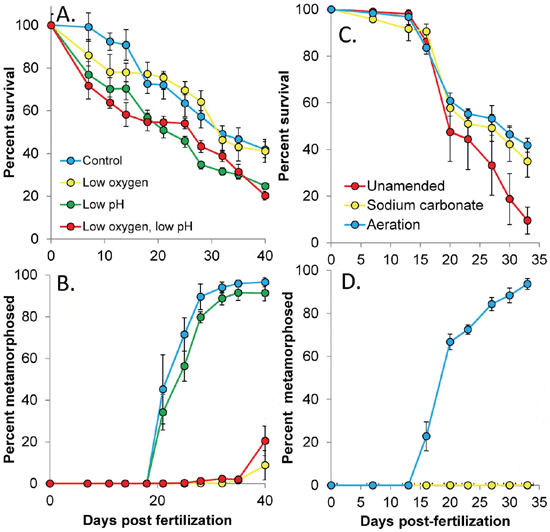
Metamorphosed individuals from normal oxygen treatments at normal and low pH conditions measured 1,130±16.8 μm and 996±36.7 μm in length, respectively, whereas metamorphosed individuals from low oxygen treatments at normal or low pH conditions were only 445±32.0 and 447±35.0 μm, respectively. Individuals that failed to metamorphose after 40 d in these treatments were even smaller (175±10.9 and 177±14.1 μm; Figure 4A).
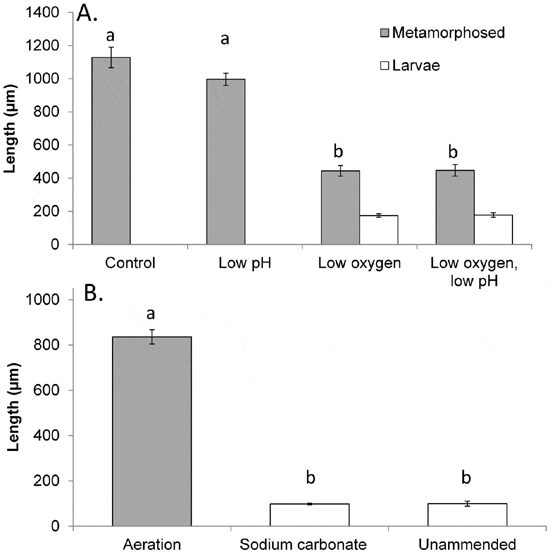
Water from Forge River Estuary used for experiments had levels of dissolved oxygen (140±56 μM), pHT (7.37±0.21), carbonate ion (1569.3 μM), and aragonite saturation (0.23±0.15) that were all significantly lower than the levels produced via the aeration of this water (260±22 μM, 7.91±0.08, 120±24 μM, 1.89±0.15, respectively; p<0.05; Figure 2B; Table S2). Aeration did not alter the alkalinity of the water (1848±119 μM for aerated and unamended treatments). The addition of sodium carbonate created water with levels of pHT (7.93±0.08), carbonate ion (98±14 μM), alkalinity (2360±186 μM), and aragonite saturation (1.51±0.24) that were all significantly greater than the unamended Forge River water (p<0.05; Figure 2B; Tables S2), but this treatment did not significantly alter levels of dissolved oxygen (142±57 μM; Figure 2B; Tables S2).
Exposure of larval scallops to estuarine waters that were naturally acidified and low in oxygen caused low rates of growth, survival, and metamorphosis, while raising pH or oxygen levels significantly improved different aspects of their performance. For example, while only 10 per cent of larvae survived a 33 d exposure to water with naturally low pH and low oxygen (Figure 2B), the addition of sodium carbonate (pH increase) and aeration (oxygen and pH increase) each significantly increased larval survival , four-fold (to ~40 per cent; Figure 3C; p<0.005; Table S8). During the month long experiment, population mortality rates were 3.2, 2.2, and 2.1 per cent d-1 in the unamended, carbonate addition, and aeration treatments, respectively. In contrast, aeration alone significantly increased the size and metamorphic rates of scallop larvae, while sodium carbonate alone did not (Figure 3D, 4B; p<0.001; Table S9, S10). Specifically, while >95 per cent of surviving individuals in aerated treatments had metamorphosed after 33 d, 0 and 10 per cent had in the unamended and sodium carbonate treatments (Figure 3D). Similarly, while individuals that were aerated had metamorphosed and grown to 835 μm in length after 33 d, those in the unamended and sodium carbonate treatments had not metamorphosed and were
Hypoxia and acidification had contrasting and age-dependent effects on juvenile hard clams (M. mercenaria). In experiments with early stage juveniles (two-month old), levels of dissolved oxygen were significantly lower in the hypoxic and combined low oxygen and acidified treatment (51.5±6.86 μM) relative to the other treatments (262±6.86 μM), while levels of pH were significantly lower in the acidified and combined low oxygen and acidified treatments (pHT= 7.51±0.01) relative to the other treatments (7.88±0.04; p<0.05; Figure 2a). These lower pH levels were accompanied by aragonite saturation states (0.7160.01) that were significantly lower than the control and low oxygen treatments (1.93±0.07; p<0.05; Table S3). Exposure of two-month old juvenile clams to low oxygen significantly reduced their survival (from 87 to 60 per cent; p<0.05), while low pH (Figure 2A) did not, and there was no interaction between these treatments (Figure 5A; p<0.05, Table S11). In contrast, the low pH water significantly reduced the growth of surviving clams by 60 per cent (from 19 to 7.9 μm d-1; Figure 5B; p<0.05; Table S12), while low oxygen did not, and there was no interaction between treatments (Table S12).
In experiments with later stage juvenile clams (four months old), levels of dissolved oxygen were significantly lower in the hypoxic and combined low oxygen and acidified treatment (44.3±4.31 μM) relative to the other treatments (28±64.31 μM) while levels of pH were significantly lower in the acidified and combined low oxygen and acidified treatments (pHT= 7.51±0.01) relative to the other treatments (7.96±0.03; p<0.05; Figure 2a). These lower pH levels were accompanied by aragonite saturation states (0.70±0.01) that were significantly lower than the control and low oxygen treatments (2.08±0.14; p<0.05; Table S4). Four month old clams were more resistant to low oxygen and pH conditions, experiencing high survival in all treatments (>95 per cent). Although their growth rates were not altered by either low oxygen or low pH when administered alone, there was a synergistically negative interaction between these factors as the combined exposure to low oxygen and low pH reduced their growth rates by 40 per cent from 12 μm d-1 to 7.3 μm d-1 (Figure 5C; p<0.05; Table S13).
Discussion
Regions of the world’s oceans with low levels of dissolved oxygen and pH have become increasingly common and will continue to expand in the near future, yet the concurrent effects of these two stressors on marine life are largely unknown. Acidification has been shown to inhibit the performance of many calcifying invertebrates as well as some vertebrates, including fish. Our findings suggest that the true impact of low oxygen zones on marine life likely requires re-evaluation, as most laboratory studies to date have manipulated oxygen with by administering nitrogen gas resulting in concurrent basification rather than acidification (Figure 1). Given that this approach misrepresents natural marine ecosystems (Figure 1), it may mask the true effects of low oxygen zones on marine animals. For example, while low oxygen severely delayed the metamorphosis and greatly restricted the growth of larval scallops, their survival was only compromised when they were concurrently exposed to the acidified conditions. While juvenile clams responded differently to hypoxia and acidification, the full effects of these processes could not have been predicted by considering only one of these treatments alone. For example, while early stage juvenile clams suffered enhanced mortality under hypoxic conditions, their growth rates were depressed by acidification. More surprising was the synergistically negative effect of concurrent acidification and hypoxia on the growth rates of late stage clams, which was not detected in either individual treatment. While the two species of bivalves studied here have been shown to be differentially susceptible to acidification, the present study demonstrates that they also respond differentially to hypoxia and acidification. As such, it is likely that making broad predictions regarding the response of ocean animals to these stressors will prove challenging.
Prior ecosystem studies investigating the distribution of coastal marine organisms within low oxygen zones have often not measured pH or carbonate chemistry and have interpreted findings solely through the lens of oxygen concentrations. By not concurrently considering acidification within these systems, the processes driving distribution patterns may be misinterpreted. In contrast, a recent open ocean study of oxygen minimum zones (OMZs) found that pteropods migrating into OMZs are more resistant to acidification than those that do not, suggesting that acidification may be as important as oxygen in shaping the distribution of these organisms within and around these zones.
Recent research has robustly demonstrated the sensitive nature of early life stage bivalves to the levels of ocean acidification projected for the end of this century. Concurrently, it has been established that some eutrophic coastal waters are already experiencing seasonal acidification. Our novel experiments with water naturally low oxygen and low pH demonstrate that these conditions negatively impact larval shellfish. For example, consistent with our experiments using mixed gases, increasing the pH of acidified coastal waters with sodium carbonate significantly improved larval survival, thereby suggesting that contemporary acidification in eutrophic coastal zones may already be reducing the survival of bivalve larvae and perhaps other organisms. Water used for experiments had levels of pH and pCO2 (7.36±0.21 and 2620±781 μatm, respectively; Table S2) that are projected to occur in open ocean surface waters under ‘business-as-usual’ scenarios in hundreds of years. As such, eutrophic coastal zones may be relatively less impacted by future atmospheric CO2 driven acidification than open ocean environments but may also provide early insight into the manner in which future climate change may shape the distribution and abundance of ocean animals.
Interestingly, mean oxygen levels during our experiment with eutrophic coastal water were low (<140 μM) but higher than levels achieved during our mixed gas experiments, yet they still reduced the size and delayed the metamorphosis of larval scallops. Given that larval bivalves with extended metamorphosis times or of smaller sized are susceptible to higher cumulative predation rates, mortality rates of larvae exposed to low oxygen conditions in the wild may be even greater than those observed during our experiments. Such elevated mortality among early life stages of bivalves would be expected to influence the distribution and abundance of adult populations. While ammonium in the Forge River was not at levels known to be toxic (<25 μM), additional stressors present in hypoxic waters such as sulfides may have further inhibited the performance of scallop larvae. Hence, future research regarding the effects of hypoxic zones on marine life may need to consider stressors beyond oxygen and pH such as elevated sulfides, ammonium, and temperature.
Our findings coupled with prior studies provide insight regarding the differing physiological mechanisms by which hypoxia and acidification may impact early life stage bivalves. Marine invertebrates commonly adapt to environmental stressors by reducing their metabolic rate, and over an extended period of time this can translate in lowered growth rates and smaller sizes. Accordingly, acidification and hypoxia both contributed toward smaller individuals in the experiments presented here. In a manner consistent with prior studies, it was exposure of larval stages to low oxygen, however, that had the largest negative effect on the growth of individuals. Larvae also experienced delayed metamorphosis suggesting these processes are linked and that the lower metabolic state induced by hypoxia does not afford larvae the aerobic scope to initiate metamorphosis. Interestingly, larvae exposed to hypoxia (but not anoxia) commonly do not suffer enhanced mortality, suggesting that the physiological response of reduced metabolism, growth, and metamorphosis are sufficient for sustaining individuals during extended hypoxia, at least during the larval stage.
Regarding acidification, research has demonstrated that lowered pH and the associated reduction in carbonate ion availability can impact multiple physiological pathways in bivalves beginning with calcification. Lowered rates of calcification can have cascading effects on larval physiology, inhibiting other biochemical processes such as RNA synthesis and lipid content as well as more general indicators of metabolism such as growth and size. For some species of bivalve larvae, including A. irradians examined here, exposure to undersaturated levels of calcium carbonate during their first week of development can elicit depressed rates of survival. Consistent with this finding, the largest differences between survival in acidification and normal pH treatments were observed during exposure of larval stage bivalve to acidification. Hence, simply lowering metabolic rates may not be a sufficient physiological response to overcome the compromised calcification associated with acidification in the early stages of some larval bivalves.
There may be a physiological feedback and synergy between low oxygen and low pH in marine invertebrates. As environmental acidification and low oxygen slow metabolic rates, internal gas exchange may slow, intracellular oxygen levels may be reduced, and CO2 from respiration may accumulate, potentially exacerbating hypercapnia and thus further lowering metabolism. This is consistent with our finding that the combination of low oxygen and low pH, but not either factor alone, inhibited the growth of the later stage hard clams. Finally, our results suggest that, in a manner consistent with the age-dependent effects of ocean acidification on early life stage bivalves, the effects of hypoxia and acidification on early life stage bivalves are also age dependent. While larvae exposed to both stressors experienced, up to 75 per cent reduction in survival, early stage juvenile survival was more modestly reduced by the two stressors (20 per cent). The two stressors combined only reduced growth (by 20 per cent) not survival, in later stage juveniles.
Hypoxia and acidification are increasingly co-occurring in the ocean, and understanding the effects of such climate change stressors on marine animals is a significant challenge. In low oxygen regions of the ocean, the tissue demand for oxygen of marine animals is often not met by ambient supply, and thus the ability of these organisms to tolerate secondary stressors such as acidification could be compromised. Thus, it is possible that even animals insensitive to acidification alone could be negatively affected by low pH when concurrently exposed to hypoxic conditions. Given that fish are generally more vulnerable to low oxygen than mollusks such as the bivalves studied here and that some early life stage fish have already been shown to be sensitive to acidification, the dual effects of hypoxia and acidification on marine teleosts may be profound.
Because hypoxia threatens fisheries and biodiversity in coastal ecosystems, environmental regulatory agencies often set management goals for dissolved oxygen to protect estuarine animals based on levels known to negatively affect marine life (e.g., 2 mg L-1 O2). Given our finding that concurrent acidification and low oxygen significantly and independently depressed growth and survival rates of estuarine bivalves, it is plausible that coastal managerial criteria based strictly on oxygen levels, but not pH, may not adequately protect marine life in some ecosystems. Therefore, future environmental regulations developed to protect estuarine organisms in regions prone to hypoxia should consider the concurrent effects of acidification on these animals, particularly as climate change accelerates the intensity of acidification in coastal zones.
The expansion of hypoxic zones in coastal and open oceans is one of many predicted consequences of intensifying global climate change. While microbial processes will continue to promote acidification in these regions, this will be exacerbated by the loading of anthropogenic CO2. We suggest that recently discovered high CO2 sensitivities in many finfish and shellfish, and the compounded effects of high CO2 and low O2 in bivalves relative to each individual parameter should prompt a re-alignment of future studies. A comprehensive evaluation of the combined effects of low oxygen and acidification on marine life is critical for understanding how ocean ecosystems respond to these conditions both today and under future climate change scenarios.
April 2014