Introduction
Methylmercury (MeHg) is a potent neurotoxin that readily bioaccumulates and biomagnifies in coastal marine food webs, which are important pathways to human mercury (Hg) exposure. While it is not presently known how climate change will affect the fate of MeHg in marine ecosystems, climate related factors, specifically rising temperatures, could increase the production and bioaccumulation of MeHg at the base of marine food webs. This, in turn, could affect human exposure through consumption of marine fish. Studies of human populations have shown correlations between total mercury (THg) in fish consumed and increased blood pressure, myocardial infarctions and delays in neurobehavioral development in children. In the US, 50 states have instituted fish consumption advisories pertaining to Hg, and coastal advisories exist for all states bordering the Atlantic and the Gulf of Mexico due to the rising concern regarding health risks associated with Hg exposure through consumption of seafood. In this study, we investigated the effect of temperature on MeHg bioaccumulation in killifish, important and ubiquitous estuarine forage fish, in both the laboratory and in the field.
Marine and estuarine systems are subject to sustained multiple stressors that include climate change and atmospheric deposition and chemical inputs of heavy metals from industry and fossil fuel emissions. The effects of climate change are particularly apparent in coastal ecosystems, as they are exposed to climate extremes. These systems are also used extensively by humans and are venues of exposure for a variety of toxic chemicals. Coastal and estuarine sediments are repositories for Hg, receiving both atmospheric and watershed inputs. Due to the biogeochemical conditions that stimulate methylation of inorganic Hg by sulfate-reducing bacteria, estuaries and coastal zones are hot spots for the transformation of Hg into its more toxic form of MeHg. MeHg produced in coastal zones is available to estuarine food webs that are important pathways to human exposure, as the consumption of marine fish and shellfish are the dominant source of MeHg to humans. The production of MeHg in coastal zones may account for much of the bioaccumulation in marine fish, along with methylation in the water column.
MeHg production and bioaccumulation in aquatic systems is affected by biological and geochemical factors. MeHg concentrations in fish and shellfish species are influenced by sediment type and biogeochemistry, which affect methylation rates and MeHg flux to the water column, and water chemistry. Climate-induced changes in environmental parameters such as dissolved organic carbon, pH, and temperature can directly or indirectly alter organismal MeHg concentrations through altered sediment metal-binding capacity, increased or decreased methylation rates, microbial activity levels, diet (benthic and pelagic community composition), and fish physiology. While climate warming and its role in altering heavy metal burdens in fish and shellfish has received attention, forecasting the effects of climate warming on MeHg bioaccumulation is difficult due to the multiple processes that influence MeHg production, bioavailability and uptake.
The Northeast US is clearly warming with rising air and sea temperatures and a lengthened growing season. In Northeast coastal waters, annual mean temperature has risen by ~1°C since the 1970’s and by 1–2°C during the summer. Predicted increases in water temperature for temperate waters are between 1.5°C and 4.5°C, making an understanding of the effect of temperature on contaminant uptake of particular interest. Approaches to predicting the effect of climate warming on heavy metal burdens have focused primarily on decadal correlations between nutrient concentrations, warmer water temperatures and metal concentrations. While reported studies on the effect of temperature on MeHg bioaccumulation are rare, particularly in marine environments, in aquatic systems, there is generally a positive correlation between warmer water temperatures and accumulation of heavy metals. Laboratory studies relating temperature to Hg and MeHg bioaccumulation in freshwater zooplankton indicate temperature may increase MeHg accumulation. Seasonal field studies suggest increased Hg methylation in sediments at warmer temperatures, which could lead to greater concentrations of bioavailable MeHg. Freshwater arctic fish have shown increasing contaminant burdens despite decreasing atmospheric deposition, with climate and temperature effects hypothesized to be driving factors for the increased exposure. These and other studies suggest that warmer temperatures may lead to greater heavy metal bioaccumulation in marine ecosystems e.g. Mechanistically, rising water temperatures can lead to increase feeding rates in response to higher metabolic demand. Higher consumption could result in greater MeHg uptake and accumulation; conversely increased consumption could also result in greater growth, leading to reduced MeHg bioaccumulation through somatic growth dilution. Given that the global climate is warming and that temperature is increasing at a more rapid rate in temperate regions than previously recorded, it is critical to evaluate the relationship between temperature and Hg bioaccumulation in temperate coastal systems. No prior studies have linked climate warming to MeHg bioaccumulation using both laboratory and field related studies. This study provides important insight into the fate of MeHg in marine ecosystems under climate warming scenarios.
We coupled field and laboratory studies to investigate the effect of temperature on MeHg accumulation in the killifish, Fundulus heteroclitus. Small fish that reside in estuaries are valuable bioindicators for MeHg uptake in local food webs because their low trophic position links them to MeHg production and entry of MeHg into marine food webs. Both their trophic position and their widespread geographic distribution make killifish a good focal species with which to examine effects of climate warming on MeHg in coastal and marine ecosystems. Temperature induced changes in killifish MeHg bioaccumulation in field studies can result from increased MeHg production and subsequent trophic transfer of MeHg through the food web and/or temperature mediated effects on fish growth. In our study, we examined killifish accumulation of MeHg in natural saltmarsh pools spanning a temperature gradient, investigated the effects of temperature alone on MeHg bioaccumulation in laboratory experiments, and evaluated possible mechanisms of temperature effect on MeHg bioaccumulation.
Results
In the field studies, temperature was the only significant term in the multiple regression for MeHg concentrations with an adjusted r2 value of 0.72 (Table 1). There was no relationship between sediment %MeHg and temperature (MS = 1.89, F =3.66, P = 0.088, adjusted r2 =0.21; Fig. 1). Pools exhibited a range of values for selected environmental variables (Table 2). MeHg concentrations in sediments ranged from 0.001 to 0.214 ng g21 MeHg in 2009 and 0.19 to 0.41 ng g21 MeHg in 2010. Percent sediment TOC ranged from 3.96 to 8.70% in 2009 and 1.03 to 10.28% in 2010. Mean salinity, temperature and dissolved oxygen values in pools ranged from 26.8 to 28.0 PSU, 18.28 to 20.87uC, 4.04 to 5.81 mg/L in 2009 and 29.0 to 31.0 PSU, 19.31 to 22.04uC, 4.72 to 5.84 mg/L in 2010 (Fig. 2). No significant differences in stable isotope values of killifish were found among pools (Fig. 3), indicating that all fish were feeding on similar prey items.
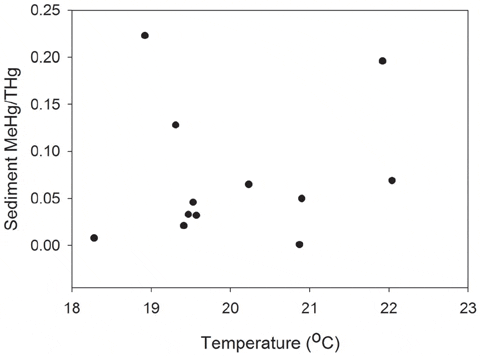
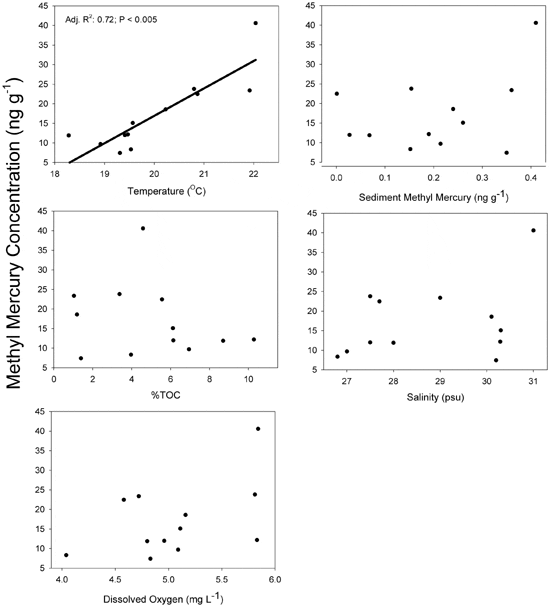
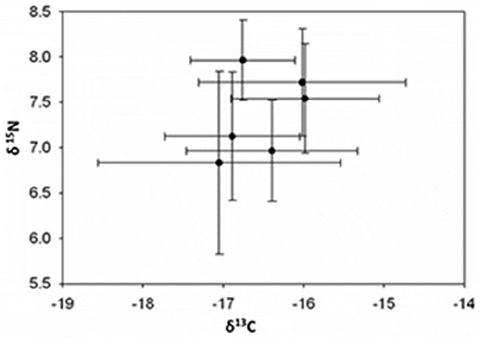
In laboratory experiments, we found a significant main effect of temperature on bioaccumulation of MeHg (Mean Squares (MS) =0.02702, F2,2= 10.13, P,0.001; Fig. 4) with greater concentrations of MeHg in fish exposed to the highest temperature, 27°C (P,0.05, Tukey-Kramer). No significant effects of trial or interaction between trial and temperature were observed (Sum of Squares (SS) =0.0007, F1,2 =0.269, P= 0.610; SS =0.0040, F2,2 =0.743, P= 0.487). Percent change in weight for each treatment was significantly different between trials, with slow or no growth in trial 2 (MS = 1927.8, F1,2= 27.7, P,0.001; Fig. 5). No effect of trial or interaction of trial and temperature were found (SS = 299.5, F2,2= 2.99, P= 0.070; SS = 295.9, F2 = 2.96, P =0.072). One-way ANOVA revealed a significant difference in the initial sizes of killifish for the two trials (MS =1.94, F1,2 =19.24, P,0.001) with smaller initial sizes of trial 1 fish (0.921 g±075) than trial 2 fish (1.439 g±091).
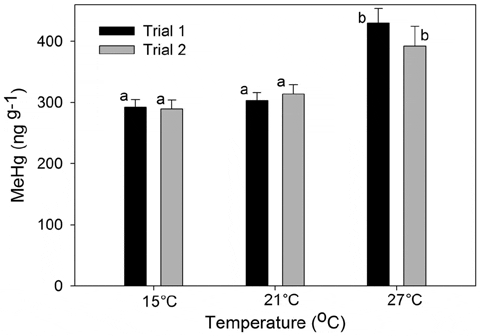
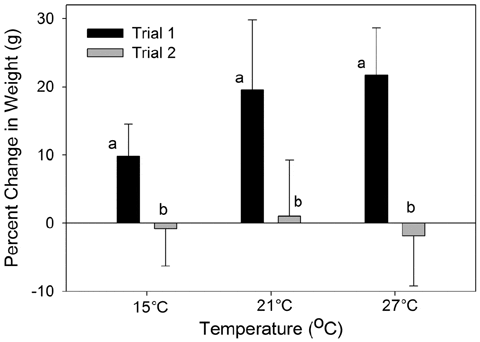
Bioenergetics Model
The proportion of maximum consumption (P) needed to produce the observed weight change during the 30 day laboratory averaged 19±2% with an estimated consumption that varied between 0.018 g g-1d-1to 0.048 g g-1d-1. MeHg assimilation efficiency (AE) from modeled consumption and fitted P values were similar across temperatures (mean values of 56%, 51% and 70% at 15°C, 21°C, 27°C). Based upon observed growth rates, the model indicated that temperature increased consumption in fish from both trials, but respiration also increased. Thus, assimilated MeHg concentrations were a function of low net relative growth of new tissue at warmer temperatures. The model indicates a possible metabolic mechanism for increased bioaccumulation: fish at the highest temperature ate more food (and thus ingested more MeHg) but grew less, leading to an increase in MeHg relative to fish at lower temperatures.
Discussion
Our field and laboratory results indicate that warmer temperatures were linked to greater bioaccumulation of MeHg in the resident estuarine killifish, Fundulus heteroclitus. Killifish have a broad geographic range and are important prey for predatory fish that move into estuaries to feed and are harvested for human consumption. F. heteroclitus spend their entire lives in a single estuarine environment and accumulate MeHg through dietary uptake of zooplankton and small benthic invertebrates. Across marine food webs, concentrations of MeHg that enter at the base of food webs will be propagated to higher trophic level species. Thus, climate-induced increases in lower trophic level bioaccumulation will likely increase human exposure to MeHg.
Effects of temperature on MeHg bioaccumulation in killifish can follow from changes in fish metabolism, prey species composition, environmental conditions influencing sediment biogeochemistry, or MeHg production and bioavailability. Killifish consume a wide range of benthic and pelagic prey including detritus, insects, amphipods and copepods. Our field study indicates fish collected from different pools had similar diets, inferred from stable isotope values, suggesting that prey preference had little influence on MeHg bioaccumulation. The duration of exposure (i.e., field fish were exposed for multiple months as opposed to 30 days in the lab) to Hg did not qualitatively change the relationship of higher temperature and increased MeHg bioaccumulation. Therefore, the observed differences in magnitude of MeHg accumulation between field and laboratory experiments can be better explained by the consumption of MeHg enriched food by laboratory fish relative to natural levels in the field.
Warmer temperatures can influence both the physiology and metabolism of fish. Fish experiencing warmer temperatures typically have elevated metabolic rates, but consumption rates may increase or decrease depending on the changes in behavior and prey availability. The effect of temperature on MeHg accumulation depends largely on growth efficiency, which integrates the effects of consumption and metabolism. The bioenergetics model based on our laboratory results suggested that killifish consumption increased with temperature, but respiration increased even more, leading to low growth, low growth efficiency, and greater MeHg bioaccumulation. The model also predicted that killifish tissue growth is faster at lower temperatures due to reduced respiration rates. Faster growing killifish should have reduced MeHg concentrations than slowgrowing fish due to somatic growth dilution. Given this, one might have expected the 27°C treatment from trial 2, which exhibited no growth, to have slightly elevated MeHg tissue concentrations than their trial 1 counterpart. This was not evident in the laboratory data and may be due to the duration of the experiment being too short for this difference to be evident in the fish (MeHg had not reached a steady state concentration). Alternatively, the highly MeHg enriched food source masked subtle effects of growth on bioaccumulation within a given temperature treatment. For natural populations, fish experiencing warmer temperatures during their growing season may have relatively increased body burdens of MeHg due to the effects of temperatures on their metabolism. However, this may be mediated or exacerbated by other environmental variables.
In our laboratory study, percent mass gain or loss in killifish differed between the two laboratory trials. Fish generally did not grow or lost mass in the second trial but not the first. The difference in growth between the trials was likely an allometric effect; average initial sizes were larger for killifish used in the second trial. Nonetheless, both trials resulted in significantly greater average MeHg concentrations in killifish exposed to the highest temperature, suggesting that the effects of temperature on growth efficiency were consistent across trials despite the overall difference in growth. There was no difference in MeHg concentrations between the low and middle temperature treatment in either trial, contrasting with the apparently linear effect of temperature in the field experiment. This difference may be due to the fact that fish had been housed at 21°C since their fall collection prior to the experiment; thus, only fish in the highest temperature treatment experienced an overall increase in temperature. We hypothesize that had the initial cohort been housed for long-term care at 15°C, both the 21°C and 27°C treatments would have indicated a significant increase in MeHg. Optimal growth for killifish that reside in the Western North Atlantic occurs at temperatures ranging from 24°C to 29°C and lethal temperature is above 36°C.
In addition to affecting fish physiology, increased temperatures could also alter environmental conditions that would change the bioavailability of MeHg to fish through changes in sediment biogeochemistry. In general, the relationship of temperature to MeHg production and flux are poorly understood for marine ecosystems. Past studies indicate that elevated temperatures in mesocosms and in the field could result in sulfate reduction rates, which could lead to higher methylation rates and greater flux to the water column. Though microbial activity was not explicitly examined in this study, there was no relationship between sediment MeHg and temperature. Past studies have used sediment MeHg/Hgt ratio (%MeHg) as a measure of methylation efficiency. Field data indicated that sediment MeHg concentrations as well as %MeHg differed slightly between pools, but these differences were not related to temperature (Fig. 1) or to fish MeHg tissue (Fig. 2). In this study, MeHg water column concentrations were not measured; while it can be inferred from the lack of relationship between temperature and sediment %MeHg that temperature had no effect on methylation rates and MeHg bioavailability to fish, it is not shown empirically in this study.
Field data in this study also indicate that MeHg bioaccumulation did not statistically relate to salinity, TOC, or dissolved oxygen, all of which can affect metal bioavailability. However, between-year variation of MeHg bioaccumulation in the field results may have been caused by temporal variation in microbial activity of sulfate-reducing bacteria, or changes to the prey community structure, salinity and ecosystem productivity. The relationship between salinity and MeHg accumulation is still unclear as studies report both a positive and a negative relationship with increasing salinity and are typically based on aqueous rather than dietary exposure. Our average difference between years (~4 psu) would not have likely affected MeHg concentrations as a recent study demonstrated there was little difference in MeHg concentrations in killifish exposed to experimental salinities ranging from 0 to 25 psu. The field results of this study suggest that across the range of environmental variables, MeHg concentration differences in killifish were only correlated with temperature (Fig. 2). The most parsimonious explanation for these results is provided by the bioenergetics model of our laboratory fish study that indicated that fish exposed to higher temperatures ate more and grew less than fish exposed to lower temperatures. This is consistent with other studies that indicate slow-growing fish have higher MeHg concentrations than fast-growing fish.
Climate change encompasses multiple changes in physical factors (e.g., wind flows, atmospheric circulation, and precipitation) that will likely affect mercury deposition and bioavailability, and biological factors (e.g., species biogeographic patterns, composition) that affect food web structure and in turn bioaccumulation. We have shown that under current predicted changes in climate warming, MeHg concentrations significantly increased under controlled laboratory and natural field mesocosm studies. However, other climate related factors would likely indirectly affect contaminant bioaccumulation in resident fish. Earlier estuarine ice-out dates and earlier winter/spring high river flows will result in longer ice-free seasons that can lengthen the growing season of killifish and affect its growth efficiency in addition to average summer temperatures. Moreover, changes in nutrient loading in estuaries due to changes in precipitation could also alter estuarine trophic status thereby changing MeHg production and bioaccumulation. Nixon et al have shown a reduction in overall productivity in Narragansett Bay with long term changes in climate and reduced productivity in freshwater systems has been shown to result in increases in MeHg bioaccumulation.
While many of these factors have the potential to indirectly alter MeHg bioavailability to marine food webs, the predicted increases in temperature will directly increase MeHg bioaccumulation at lower trophic levels such as killifish. This increase can be propagated to higher trophic level fish consumed by humans, resulting in increased human exposure to MeHg. This effect should be incorporated into policy and management efforts aimed at reducing human health risks from MeHg exposure.
Acknowledgments
We would like to thank the following people for their assistance with field and laboratory research; Curtis Hansen, Deenie Bugge, Hannah Roebuck, and Jaqueline Boudreau. We would also like to thank Kimberly Lellis- Dibbles for processing and running our samples for stable isotope analysis.
Author Contributions
Conceived and designed the experiments: JAD KLB DW CYC. Performed the experiments: JAD KLB MD. Analyzed the data: JAD KLB MD. Contributed reagents/materials/analysis tools: DWE. Wrote the paper: JAD KLB CYC DWE MD DW.
January 2014