Although this use of fishmeal was initially the recycling of waste from fishing through the use of bycatch and trimmings, due to the rapid development of aquaculture this reliance on fishmeal and fish oil is environmentally unsustainable. This has resulted in other sources of fish feed being investigated. This literature review will focus on microalgae; the composition in terms of nutritional quality, the current methods of production and associated costs along with potential future uses such as feed in aquaculture.
Algae overview
Marine algae are distributed from the polar regions to tropical seas in nutrient rich and poor environments. Algae are photoautotrophs and are characterised by their lack of roots, leaves and presence of chlorophyll a. They range in size from microscopic individual cells called microalgae to seaweeds that can be greater than 30m in length (Qin 2012).
Marine microalgae are the dominant primary producers in aquatic systems and account for a similar level of carbon fixation as terrestrial plants (40-50 per cent) but represent only 1 per cent of the planetary photosynthetic biomass (Stephenson 2011). Microalgae are sometimes directly consumed by humans as health supplements due to this high nutritional value and abundance (Dallaire 2007) but this is relatively rare.
As carnivorous fish ingest algae as a food source (Nakagawa 1997) there has been a move to utilise them for fish feed. Currently 30 per cent of the world algal production is used for animal feed (Becker 2007) but the use in aquaculture is mainly for larval fish, molluscs and crustaceans (FAO 2009a). As mentioned above the fishmeal and oil use in aquaculture is unsustainable and algae have the potential to reduce this dependence. This is due to the algae being photosynthetic so have the ability to turn the sun’s huge amount of energy, 120,000 TW of radiation, into protein, lipids and nutrients. More energy from the sun hits the surface of the earth in one hour than the energy used in one year and this is a huge amount of untapped, sustainable energy can be exploited by algae. This is a relatively new area of research but has many positive aspects that give it a large amount of potential for future use.
Microalgae
The term ‘microalgae’ is often used to refer specifically to eukaryotic organisms, both from freshwater and marine environments but can include prokaryotes such as cyanobacteria (Stephenson 2011). Microalgal production has received some attention recently due to its potential use as a biofuel (Slocomb 2012), use in animal feed, human consumption and recombinant protein technology (Becker, 2007; Potvin and Zhang 2010; Williams and Laurens, 2010). This has resulted in a huge amount of knowledge and research into microalgae and resulted in reviews being published about specific subjects such as genetic engineering of algae (Qin 2012), potential use as biofuel (Demirbas 2011) and novel methods to measure such important components such as protein (Slocomb 2012).
This interest and knowledge in the area has allowed aquaculture to essentially piggy back the research being performed by the biodiesel industry and even act synergistically with it by consuming the by-products produced (Ju 2012).Currently microalgae have been used in aquaculture as food additives, fishmeal and oil replacement, colouring of salmonids, inducing biological activities and increasing the nutritional value of zooplankton which are fed to fish larvae and fry (Dallaire 2007).
Although the biodiesel industry has been conducting a large amount of research this has mainly been focused towards species that have high lipid contents whereas species in aquaculture must be of appropriate size for ingestion and be readily digested. They must also have rapid growth rates, be able to be cultured on a mass scale, be robust enough to cope with fluctuations in temperature, light and nutrients and have a good nutrient composition (Brown 2002).
Varying nutritional values
The nutritional value of any algal species depends on its cell size, digestibility, production of toxic compounds and biochemical composition. This, along with differences among species and method of production, explains the variability in the amount of protein, lipids and carbohydrates, which are 12-35 per cent, 7.2-23 per cent, and 4.6-23 per cent respectively (FAO 2009a) (Figure 1). This level of fluctuation can be influenced by the culture conditions (Brown et al., 1997) but rapid growth and high lipid production can be achieved by stressing the culture.
Protein
Most of the figures published in the literature on the concentration of algal proteins are based on estimations of crude protein and as other constituents of microalgae such as nucleic acids, amines, glucosamides and cell wall materials which contain nitrogen; this can result in an overestimation of the true protein content (Becker 2007).
The non-protein nitrogen can be up to 12 per cent in Scenedesmus obliquus, 11.5 per cent in Spirulina and 6 per cent in Dunaliella. Even with this overestimation the nutritional value of the algae is high with the average quality being equal, sometimes even superior, to conventional plant proteins (Becker 2007) (Table 1).
The amino acid composition of the protein is similar between species and is relatively unaffected by the growth phase and light conditions (Brown et al., 1993a, b). Aspartate and glutamate occur in the highest concentrations (7.1-12.9 per cent) whereas cysteine, methionine, tryptophan and histidine occur in the lowest concentrations (0.4-3.2 per cent) with other amino acids ranging from (3.2-13.5 per cent) (Brown 1997).
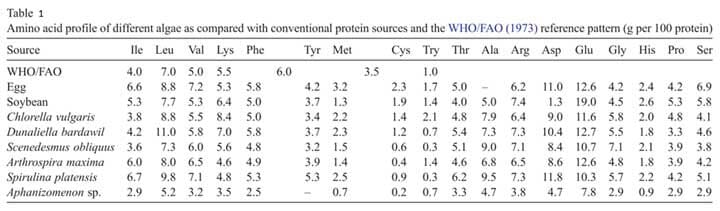
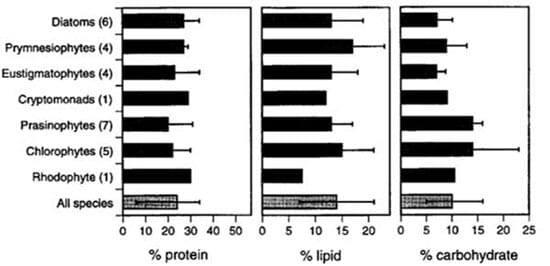
Lipids
The lipids in microalgal cells have roles as both energy storage molecules and in the formation of biological membranes and can be as high as 70 per cent dry weight in some marine species (Stephenson 2011) (Table 2). Under rapid growth conditions these lipid levels can drop to 14-30 per cent dry weight, which is a level more appropriate for aquaculture. These lipids are composed of polyunsaturated fatty acids such as docosahexaenoic acid (DHA), eicosapentaenoic acid (EPA) and arachidonic acid (AA) (Brown 2002) and in high concentrations; most species have per centages of EPA from 7-34 per cent (Brown 2002) (Figure 2).
These fatty acids are highly sought after and as they currently cannot be synthesised in a laboratory are usually obtained through fish oil and are a limiting factor in vegetable oils such as palm, soybean and rapeseed oil use in aquaculture. The fatty acid composition is associated with light intensity, culture media, temperature and pH. Appropriate measures and control, along with the suitable selection of a species, is necessary to produce algae with the desired lipid level and composition.
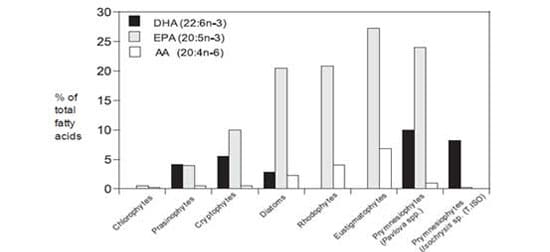
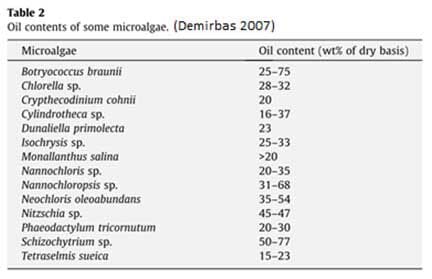
Vitamins
Microalgae also contain vitamins which can be beneficial to the health of the consumer but vary greatly between species (Brown 2002). This variation is greatest for ascorbic acid (Vitamin C), which varies from 1-16mg g-1 dry weight (Brown & Miller, 1992), but other vitamins typically show a 2-4 x difference between species (Brown et al., 1999) (Figure 3).
Despite the variation in vitamin C all the species would provide an adequate supply to cultured animals which are reported to only require 0.03-0.2 mg g-1 of the vitamin in their diet (Durve and Lovell, 1982). However every species of algae had low concentrations of at least one vitamin (De Roeck-Holtzhauer et al., 1991) so a careful selection of a mixed algal diet would be necessary to provide all the vitamins to cultured animals feeding directly on microalgae.
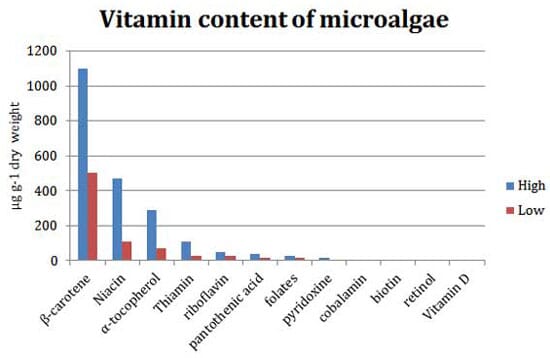
Algae in aquaculture
The use of algae as an additive in aquaculture has received a lot of attention due to the positive effect it has on weight gain, increased triglyceride and protein deposition in muscle, improved resistance to disease, decreased nitrogen output into the environment, increased fish digestibility, physiological activity, starvation tolerance and carcass quality (Becker, 2004; Fleurence 2012). Li (2009) showed that the addition of dried microalgae in the diet, albeit at low concentrations 1.0-1.5 per cent, resulted in increased weight gain of the channel catfish (Ictalurus punctatus)along with improving the feed efficiency ratio and levels of poly-unsaturated fatty acids. Ganuza (2008) showed that algal oil can be an alternative source of DHA (docosahexaenoic acid) to fish oil in gilthead seabream (Sparus aurata) microdiets although it did not allow for the complete substitution of fisheries products due to the low EPA (eicosapentaenoic acid) levels in the species of algae used.
These were at relatively low-level inclusions; at greater levels it can have a detrimental effect. At 12.5 per cent inclusion algae caused reduced growth performances in rainbow trout and at 25 per cent and 50 per cent this substitution of fish feed caused nutritional deficiencies that led to decreased growth, feed efficiency and body lipids (Dallaire 2007).
Levels of algal inclusion of 15 per cent and 30 per cent also reduced feed intake and growth rate in Atlantic cod (Walker 2011). As Atlantic cod are known to have a robust digestive system it was suggested that this was due to reduced palatability which could be an issue for algal use in aquaculture.
High levels of inclusion does not cause such negative effects in all species raised in aquaculture, 50 per cent replacement did not have a negative effect on shrimp (Ju 2012), but is generally experienced among finfish.
Algae production
The production of algae, in particular microalgae, is a rapidly developing industry due to the biofuel research that is currently taking place. The annual world production of all species is estimated to be 10,000t year-1 (Richmond, 2004) with the main limit to production currently being the cost. Production costs are currently range from US$4-300 per kg dry weight (FAO 2009a) depending on the type of production method employed (Table 3).
There has been a shift away from typical systems such as outdoor ponds and raceways to large-scale photobioreactors which have a much higher surface area to volume ratio and could potentially reduce the production cost (Brown 2002). These photobioreactors could yield 19,000 - 57,000 litres of microalgal oil per acre per year, which is over 200 times the yield from the best performing vegetable oils (Chisti 2007), and reduce the cost of algal oil from $1.81 to $1.40 per litre (Demirbas 2011).
However, for algal oil to be competitive with petrodiesel, it should be less than $0.48 per litre. This is achievable through economies of scale (Demirbas 2011) and would make it a cheap and sustainable oil for the aquaculture industry. There are also other developments such as increasing the specific activity of the enzyme RUBISCO which would increase yields, transgenic studies, increasing the proportion of photo protective pigments which would improve the light-dependant reactions and selecting for algae with small antennas which is fundamental to achieving high yields in biomass dense cultures (Stephenson 2011). This research is essential as the production costs of microalgae are still too high to compete with traditional protein sources for aquaculture (Becker 2007).
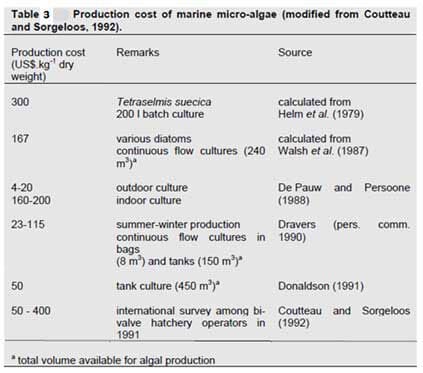
Benefits and obstacles
Algae have a great potential for use in sustainable aquaculture as they are not only a source of protein, lipids and have other nutritional qualities but they are phototrophic so produce these directly from sunlight. Producing 100 tons of algal biomass also fixes roughly 183 tons of carbon dioxide which has obvious implications in this period of climate change.
The production does not always require freshwater, compete for fertile land and are not nutritionally imbalanced with regard to the amino acid content like soybean.
There are still some obstacles such as the powder-like consistency of the dried biomass and applications to feed manufacture, the production costs and pests and pathogens that will effect large scale algal cultivation sustainability (Hannon et al., 2010), which is an area that little is known about.
There still needs to be many feeding trials as the majority of research has focused on improving the nutritional value of rotifers and not as algae as a potential replacement of fishmeal and fish oil. There is also interest into storing algal pastes which have extended shelf life (2-8 weeks) or the use of defatted microalgae meal from the biodiesel industry.
The use of algae in aquaculture is a promising and young area of research and when compared to agriculture, which has increased crop productivity by 138 per cent in a 50 year period, it demonstrates the great potential that algae has.
December 2013