The concentration of carbon dioxide (CO2) in the atmosphere is rising at a rate unprecedented for millions of years, due to the release of CO2 from fossil fuel burning, cement production, and land use changes by humans (Doney et al. 2009). Atmospheric CO2 is now 400 ppm (Dlugokencky and Tans 2013), higher than any time in the past 800,000 years (Luthi et al. 2008), and could exceed 900 ppm by the end of the century if the current emissions trajectory is maintained (Meinshausen et al. 2011; Peters et al. 2012). One of the consequences of rising atmospheric CO2 concentrations is the increased absorption of CO2 in the ocean. Here, it reacts with seawater, causing a reduction in the concentration of carbonate ions and lowering seawater pH, a process known as ocean acidification (Raven et al. 2005). The partial pressure of CO2 (PCO2) in the ocean also increases with increasing atmospheric CO2 because the ocean surface is at approximate gas equilibrium with the atmosphere (Doney 2010). These changes to ocean chemistry have been shown to affect fundamental biological processes, such as metabolism, growth, calcification, reproduction, and behavior, in a wide range of marine organisms (Fabry et al. 2008; Widdicombe and Spicer 2008; Doney et al. 2009; Kroeker et al. 2010; Briffa et al. 2012). However, the potentially interacting effects of ocean acidification on multiple biological traits, and the effects on ecological interactions among organisms, remain poorly understood (Fabry et al. 2008; Hendriks et al. 2010; Kroeker et al. 2013). Anticipating the responses of marine organisms to rising CO2 levels and ocean acidification is a crucial test case for evolutionary ecologists.
Recent studies show that exposure to elevated CO2 causes fish to fail to respond to ecologically important chemical cues including homing odors (Munday et al. 2009) and predation cues (Dixson et al. 2010; Ferrari et al. 2011a). Munday et al. (2010) and Ferrari et al. (2011b) both found that larval fish (Pomacentris wardi and Pomacentris chrysurus) raised in seawater enriched with levels of CO2 predicted for the end of this century dramatically altered their behavior and displayed higher mortality compared to fish raised in current-day seawater. Fish exposed to elevated CO2 levels have also been found to have impaired auditory abilities (Simpson et al. 2011) and reduced behavioral lateralization (Domenici et al. 2012) providing evidence that high CO2 directly affects brain function in juvenile fish (Nilsson et al. 2012). One study has demonstrated that ocean acidification will also affect recognition or cognitive processing of visual information. Ferrari et al. (2012) found that larval damselfish raised in high-CO2 seawater responded differently to the sight of a large nonpredatory fish (a spiny Chromis, Acanthochromis polyacanthus) to which the prey was unfamiliar. Fish exposed to current-day levels of CO2 reacted to A. polyacanthus with antipredator behaviors indicating that the prey may show neophobic responses to any large fish, regardless of whether they pose a threat (Brown et al. 2013). Whether ocean acidification will impair visual recognition of prey to common predators is currently unknown.
A recent study by Devine et al. (2012) found that there were differential effects of CO2 on different senses and that one sense might overcome the impairment of another sense. Such sensory redundancy could reduce the effects of high CO2. In Devine et al.’s (2012) study, three species of damselfish that depend on both olfactory and visual cues to find appropriate settlement habitats retained the ability to select their preferred habitat although their olfactory sense had been impaired. This suggests that at least some fish will rely on other senses to compensate for the loss of one sense. Here, we investigated the effects of elevated CO2 on responses of fishes to visual, chemical, and visual cues paired with chemical cues of predators. Specifically, we were interested in experimentally testing whether appropriate responses to visual information could compensate for impaired olfactory antipredator senses that are commonly reported in damselfish.
The early life stages of many animals, including fishes, are extremely vulnerable to predation (Almany and Webster 2006). Due to their development in the plankton, settlement stage reef fish arrive to habitat patches that contain predators never before encountered. At this time, individuals would benefit from possessing a preprogrammed (i.e., innate) sensory response to a predation threat, be it visual or olfactory. There are many cases of fishes displaying innate responses to skin extracts of injured conspecifics (Chivers and Smith 1998; Lonnstedt and McCormick 2011) and odor cues emitted by their natural predators (Hawkins et al. 2004; Dixson et al. 2010). However, limited research has investigated if native prey fish display an innate response to the visual cues of predators (for an exception see Coss 1979). Highly diverse environments, such as coral reefs, contain vast numbers of fish of different colors, sizes, and body shapes (Marshall et al. 2003). Here, the ability to visually discriminate between predators and nonpredators should be of crucial importance for native prey. While it is known that ocean acidification impairs the ability of prey fish to detect olfactory signposts of risk, it is unknown whether visual information may partially compensate for the lack of olfactory abilities, and thus help maintain population replenishment in acidified oceans.
The current study aimed to determine how prey fish (ambon damselfish, Pomacentrus amboinensis) respond to the separate and combined effects of olfactory and visual cues of predators when exposed to a CO2 level (880 latm CO2) projected for the surface ocean by the end of this century (Doney 2010; Meinshausen et al. 2011). We specifically chose to study ambon damselfish because they appear to be the most sensitive of the four species of damsel fishes to CO2-induced impairment of their olfactory sense (Ferrari et al. 2011b). In order to test whether appropriate responses to visual cues could compensate for the loss of response to chemical cues, we conducted three experiments. The first experiment was designed to test how native (with no prior experience of predators) fish exposed to elevated PCO2 (880 μatm) respond to damage released skin extracts of conspecifics. Secondly, we tested whether responses to visual cues of a common predator were impaired in response to elevated PCO2. The final experiment examined if fish retained an antipredator response when exposed to visual and chemical indicators of risk simultaneously, testing whether the visual sense could overcome impairment of the olfactory sense.
Results
Experiment 1: Does elevated CO2 impair responses to chemical alarm cues?
There was no effect of elevated CO2 levels on the behavior of fish during the prestimulus period (Pillai’s Trace: F6,166 = 0.08, P > 0.5). However, there was a strong influence of elevated CO2 on the response of fish to conspecific skin extracts (Pillai’s Trace: F6,166 = 0.23, P = 0.002; Fig. 1). P. amboinensis from the elevated CO2 treatment did not elicit an antipredator response when exposed to the conspecific skin extracts (Fig. 1A and B). In contrast, prey exposed to current-day CO2 treatment displayed a typical threat response to conspecific chemical alarm cues, greatly reducing both foraging (F2,84 = 11.5, P < 0.001; Fig. 1A) and activity rates (F2,84 = 5.2, P = 0.007; Fig. 1B) compared to those exposed to heterospecific skin extracts or to saltwater control. Fish exposed to either elevated or current-day CO2 treatments did not increase time spent in shelter upon the injection of conspecific skin extracts (F2,84 = 1.6, P = 0.2; Fig. 1C). Although there was a tendency for fish from the current-day CO2 treatment to spend more time in shelter following injection of conspecific skin extracts, this was not statistically significant due to the large variation in responses among individuals (Fig. 1C).
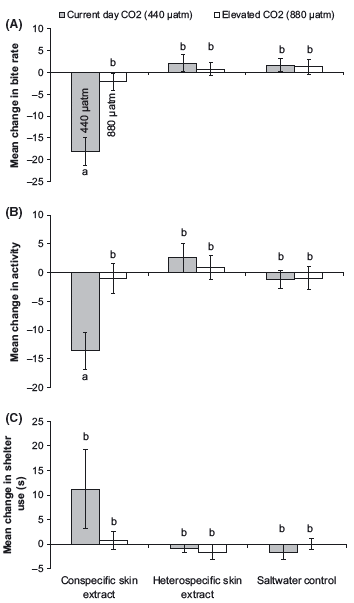
Experiment 2: Does elevated CO2 impair responses to the sight of a predator?
There was no effect of elevated CO2 levels on the behavior of fish during the prestimulus period (Pillai’s Trace: F6,166 = 0.43, P > 0.5). Overall there was no effect of elevated CO2 on the visual response of prey to the predator compared to the two controls (Pillai’s Trace: F3,164 = 0.14, P = 0.4; Fig. 2). Univariate ANOVAs revealed that there was a significant reduction in feeding rate (F2,84 = 1.3, P = 0.3; Fig. 2A) and activity (F2,84 = 0.9, P = 0.4; Fig. 2B) when P. amboinensis juveniles were exposed to the sight of a predator irrespective of the CO2 treatment. Time spent in shelter increased in fish exposed to the visual sight of a predator compared with the two experimental controls (F2,84 = 4.6, P = 0.01; Fig. 2C); however, the response was not identical between CO2 treatments. Fish from the curcurrent- day treatment significantly increased shelter use upon being presented with the visual cue of a predator control, whereas there was no significant difference in shelter use in the fish exposed to elevated CO2 levels and the two controls (heterospecific skin extract and saltwater). The mean change in shelter time of fish from the elevated CO2 treatment was intermediate to the fish from the current-day treatments and the two controls, suggesting that there were some effect of the high CO2 on visual response to the predator (Fig. 2C).
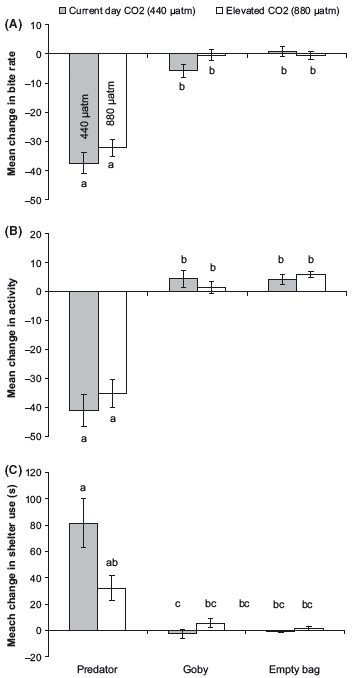
Experiment 3: Does sensory redundancy reduce the apparent impact of elevated CO2?
As observed in the previous two experiments, there was no effect of elevated CO2 levels on the behavior of fish during the prestimulus period (Pillai’s Trace: F6,166 = 0.48, P > 0.5). The MANOVA revealed that there was an interaction between CO2 treatment and cue source on the different antipredator responses of fish (Pillai’s Trace: F3,164 = 0.69, P = 0.001; Fig. 3). In fish exposed to current-day conditions, the combined cue sources gave the strongest threat responses whereas olfactory cues alone gave the weakest reaction (Fig. 3). Fish exposed to elevated CO2 concentrations did not respond to skin extracts, and the magnitude of their response to combined cue sources did not differ from that elicited when exposed to visual cues alone. Post hoc tests revealed that prey from the current-day treatment exposed to both cue sources significantly reduced foraging and activity and increased time spent in shelter compared with the CO2- treated fish (Tukey’s HSD: P < 0.05: Fig. 3).
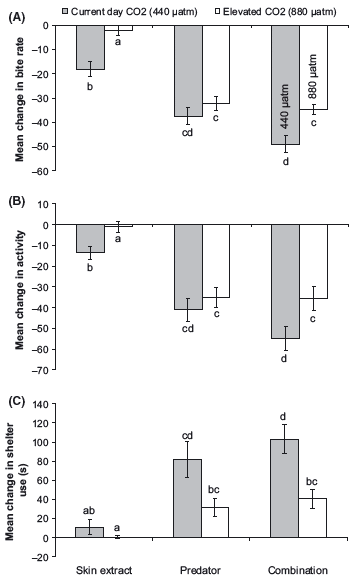
CO2 treatment and cue exposure significantly affected the outcome of frequency of bobbing behavior by P. amboinensis (Table 2; model 4 was the best fit; Fig. 4). The inclusion of CO2 treatment in the model resulted in the greatest improvement in the fit of the log-linear model (Table 2; models 1 vs. 3) compared to the inclusion of cue in the model (Table 2; models 1 vs. 2). Therefore, although the outcomes of trials were dependent on both treatment and cue type, CO2 treatment had the greatest influence on frequency of bobs in P. amboinensis. Fish in the control treatment always responded with bobbing behavior to the simultaneous exposure of both cue sources, whereas fish in the elevated CO2 treatment bobbed significantly less than control fish, with only two of 15 fish displaying this type of antipredator behavior (Fig. 4).
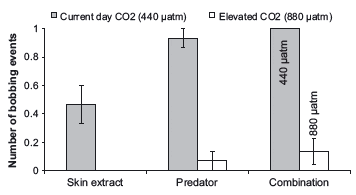
Discussion
This study suggests that both the visual and chemical antipredator systems of native prey are sensitive to changes in ocean acidification; however, the visually based behavioral responses are less affected than chemically based responses. Fish exposed to elevated CO2 completely failed to respond chemical alarm cues. While still responding to the sight of a common predator with reduced foraging and movement, P. amboinensis exposed to high CO2 displayed a delayed response to the piscivore spending less time in shelter. Furthermore, CO2-treated fish did not display the typical “bobbing” response common to damselfish when faced with a threat. It has been suggested that this “bobbing” behavior evolved as a means of pursuit deterrence; prey signal to the predator that they are aware of its presence, indicating to the predator that it is less likely to be successful in a strike (Smith and Smith 1989; Feeney et al. 2012). The lack of bobbing in fish exposed to elevated CO2 suggests that prey are capable of visually detecting a large shape, responding with increased vigilance, but may not label the shape as a predator. Alternatively, the fish could well recognize the predator, but choose not to initiate bobbing if this is an energetically costly behavior. Bobbing behavior is probably similar to that of stotting behavior in gazelles. Stotting is an honest signal of escape ability and only initiated by individuals that have the ability to escape from the predator (Caro 1986). The lack of a response to the herbivorous goby (visual control) in both the high- and low-CO2 treatments suggests that native fish are in fact able to visually discriminate between a threatening and nonthreatening fish. The response of the native prey exposed to acidified waters may simply represent a conservative neophobic response rather than an innate anti-predator response. This apparent lack of ability to correctly categorize a predator, and thus assess risk, will have ramifications for survival in encounters with predators in the field (e.g., Munday et al. 2010).
In marine environments, visual and chemical cues are the key sources of information for assessing predation risk (Brown and Chivers 2006; Lonnstedt et al. 2012). Olfaction is often the first sense to alert a prey to the presence of a potential predator and once the prey is in the direct vicinity of the stimulus source vision takes over as the primary mode of predator detection (Brown and Chivers 2006).Visual cues are more reliable as they are fast, highly directional and provide accurate information on which informed behavioral decisions can be made, including predator size, speed of movement, and direction, as well as the likelihood of attack (Coss 1979; Helfman 1989). However, to accurately assess the level of threat that a predator poses, prey will often use multiple sensory cues as visual and chemical information provide complimentary information (Lima and Steury 2005; McCormick and Manassa 2008). Despite this, only few studies have compared the relative importance and balance of more than one stimulus. Given that predator avoidance behavior is modified based on the magnitude of threat, visual information may maintain antipredator behavior, even while olfaction is impaired. Although native prey exposed to current-day CO2 levels responded slightly more strongly to the simultaneous exposure to both sensory cues compared with the fish maintained in the elevated CO2 levels, there was no significant difference in the magnitude of responses in two common antipredator behaviors (activity and foraging) compared with the experimental controls when fish from both treatments were exposed to the sight of a predator. This suggests that the visual system of prey fish may be able to help mitigate some of the effects of the loss of the olfactory antipredator system, thus decreasing prey vulnerability to predators in acidified oceans.
Animals have been found to rely more strongly on one type of cue in environments where other cues necessary for predator detection are lacking. Fish have been found to rely more heavily on their chemical sense in situations where visual cues are limited. Stronger antipredator responses are found at night (Leduc et al. 2010) in turbid waters (Hartman and Abrahams 2000; Leahy et al. 2011) and in topographically complex habitats (M. I. McCormick, unpubl. data). Similarly, Chivers et al. (2001) found that in high visibility environments fish only respond to chemical cues if given in conjunction with the visual cue of a predator. In this study, we found that visual cues may be able to help compensate for the inability of fish to recognize threatening olfactory cues as fish still responded to the sight of P. fuscus with reduced foraging and movement. In the high clarity waters of coral reefs, it is crucial for prey fish to retain a visual response as they are surrounded by a multitude of different predators.
Our findings suggest that response of native prey to the predator P. fuscus is innate. Few studies have investigated the innate recognition of prey fish to the visual cue of piscivores. Coss (1979) found that native (reared in isolation; having received no previous predator cues) African jewel fish fry (Hemichromis bimaculatus) elicit evasive antipredator behaviors when exposed to the sight of a model with two front facing eyes (believed to be a widespread signal of danger) compared to a model with no eyespots. P. fuscus similarly has two frontally placed eyes, likely to label the fish as dangerous to new settlers. Coss’s (1979) study taken together with the current study suggests that the ability of at least some fish to recognize predators appears to be predetermined. Katzenstein and Goren (2006) found that damselfish juveniles classify line drawings with “smiley faces” as nonthreatening and line drawings with “sad” faces as predators. It appears that fish are good at categorization of visual stimuli, thus it is possible that juveniles can generalize from their experiences with predators in the pelagic environment to predators on the reef. However, this visual recognition system appears to be impaired by ocean acidification as two of the crucial antipredator behaviors (bobbing and hiding) were absent in fish exposed to high-CO2 seawater. The lack of bobbing in native P. amboinensis exposed to acidified seawater suggests that the prey fail to recognize the predator as a threat, alternatively failing to initiate an appropriate antipredator response despite recognizing the piscivore as a threat, consequently spending less time in the security of shelter. This response could be attributed to CO2 interfering with the nervous system of fish. An elegant study carried out by Nilsson et al. (2012) found that similar CO2 levels (~900 μatm) inhibit the sensory system of fish by interfering with GABA-A neurotransmitters, thereby affecting chemosensory, auditory, and visual abilities. If settlement stage fish are unable to determine the degree of possible threat due to reduced cognitive abilities, they may act in a cautious manner (as they would to any new stimulus) but not with the same intensity as to the presence of a known predator, as was found in this study.
Our results imply that some antipredator behaviors of fish to chemical and visual threats will be affected by ocean acidification. While the visual response is not entirely lost, the sense is affected by rising CO2 levels. Native prey exposed to higher CO2 concentrations did respond to the presence of a predator, but with a lower intensity than control fish, failing to retire to the safety of shelter. Their lack of appropriate behavioral responses to piscivores could pose a major problem when crepuscular and nocturnal predators are active, as vision is even more limited during these hours. This is the time of the day when the majority of mortality occurs, and a well-developed visual sense along with the olfactory sense plays a key role in the identification and avoidance of predators. The cost of missing a sign of a nearby predator can be fatal, as was seen in Munday et al. (2010) were larval fish (P. wardi) raised in seawater enriched with levels of CO2 predicted for the end of this century (~850 μatm) displayed up to nine times higher mortality compared to fish raised in current-day seawater. These fish had access to all naturally available predation cues, indicating that a partially functioning visual system was insufficient to prevent dramatic increases in predation of high CO2 exposed fish. Additional field experiments are needed to determine whether the same would hold true for ambon damselfish.
Our study and previous studies have come to the same conclusion; the sensory systems and behavioral responses of fish will be severely affected in future acidified oceans. A key question is whether marine organisms will be able to adapt to the changing pH of the world’s oceans (Kelly and Hofmann 2012; Munday et al. 2012a). We already know that some animals are no longer able to cope with environments they have spent thousands of generations specifically adapting to (Walther et al. 2002). And it is a cause for concern when the nervous system and instinctive behaviors of native prey are damaged or lost as a consequence of rising CO2 concentrations. Nevertheless, some studies have detected reduced impacts when several generations are exposed to the same high CO2 environment (Miller et al. 2012; Parker et al. 2012) and there could be the potential for selection of CO2 tolerant genotypes to occur over coming generations (Munday et al. 2012b). Whether differences in the severity of impacts to different sensory systems increases the potential for adaptation to a high CO2 environment remains to be seen, but should be a priority area for future research.
February 2014