Bacteria, viruses, parasites and naturally occurring toxins in fresh and processed shellfish can cause food-borne illnesses. Shellfish also can be contaminated by substances or organisms introduced into the environment through animal and human pollution or agricultural runoff. Other factors that can increase the risk of illness include environmental conditions in the growing waters, harvesting methods, processing operations, and handling during marketing. Some shellfish present a much greater risk than others. For example, most shrimp are fully cooked before consumption, which destroys or reduces the bacteria, viruses and parasites that cause illness. The greatest risk from shrimp consumption is an allergic reaction in some people. However, many molluscan shellfish (primarily oysters and mussels) are eaten raw or with minimal heating. This is a major concern to national and international health agencies because bacteria in these products have caused food-borne illnesses and deaths. Some organisms of concern include the bacteria Salmonella, Escherichia coli, Listeria monocytogenes, Clostridium botulinum, Campylobacter, Aeromonas hydrophila, and Vibrio spp.; the virus Hepatitis A; and the parasite Cryptosporidium. Fortunately, some post-processing operations effectively eliminate or reduce the health hazards to an acceptable level.
Both the National Advisory Committee on Microbiological Criteria for Foods (NACMCF, 1990) and the International Commission on Microbiological Specification for Foods (ICMSF, 1988) have set microbiological criteria for assessing the effectiveness of Quality Assurance and HACCP programs.
Shrimp
Shrimp are the most important exported aquatic product in the global seafood market. About 75 percent of shrimp production, whether cultured or wild-caught, originates from developing countries, but 70 to 75 percent of global shrimp consumption occurs in developed countries. Shrimp is marketed cooked or raw, peeled or unpeeled, and with or without breading or other coatings. Spoilage of fresh and frozen shrimp is largely caused by the biochemical changes induced by microbial populations and, to a lesser degree, by endogenous enzymes and chemical compounds in the shrimp.
Potential Hazards in Shrimp Production
Coliforms can be found in harvested shrimp as well as in the water and sediment in which they are reared. Coliform counts in water samples may be affected by the timing of sampling with respect to water exchange on the farm, the ambient temperature at the time of sampling, and the stocking density. Levels tend to be lower if sampling coincides with water exchange, cold weather or colder water temperatures, or low stocking densities. In a pond, it is presumed that feed and manure are the major sources of Escherichia coli.
Salmonella are associated with pond water, sediment and shrimp throughout the culture cycle, including the pre-stocking period, farming phase, and harvest. The survival rate of the microorganism is enhanced by nutrients, manure and feed present in the pond system and by the favorable interaction of various biological and physical factors. Untreated chicken manure used to fertilize ponds and droppings from aquatic birds are significant sources of Salmonella.
Bacteria of the genus Vibrio are ubiquitous in all the marine and estuarine ecosystems in which shrimp are typically farmed. Several Vibrio spp. form part of the natural biota of fish and shellfish. Vibrio harveyi and V. parahaemolyticus are considered to be opportunistic pathogens that cause bacterial infections in stressed shrimp. Of the more than 20 Vibrio species known to be associated with human disease, V. cholerae, V. parahaemolyticus and V. vulnificus are of greatest concern. Clinical manifestations of these bacterial infections range from gastroenteritis to septicemia and wound infection.
Vibrio cholerae has been isolated from pond mud, water and shrimp samples in Thailand and India. The major sources of this Vibrio species in shrimp samples may be sediment, water and feed. However, natural shrimp food such as algae, plankton, invertebrate animals such as copepods, and zooplankton could also be contributors. Dalsgaard et al. (1995) studied the presence of V. cholerae in a total of 107 samples that included water, sediment, shrimp, feed, shrimp gut and chicken manure. Thirty-three percent of the samples contained V. cholerae non-01 serotype, which is less severe than V. cholerae 01, the organism that causes epidemic cholera. The occurrence of this bacterium was not significantly influenced by water, salinity, temperature, dissolved oxygen or pH.
Vibrio spp. have been found in shrimp during all phases of farming and harvest and these microorganisms are common in other crustaceans as well. Crustaceans have an exoskeleton made of chitin, and some bacteria, including Vibrio spp., can use chitin as a nutrient source. In fact, chitin has been shown to stimulate the production of cholera toxin in V. cholerae 01. In filtered water alone, all Vibrio strains produce small amounts of toxin, which indicates the need for nutrient sources to fuel this activity.
The microorganism V. parahaemolyticus has been found in shrimp from the Philippines and in shrimp pond sediment and water from Thailand. Sediment, water and feed sources are sources of the bacterium. However, the natural association between pathogenic V. parahaemolyticus and shrimp, especially with shrimp shell chitin as a nutrient source for this pathogen, should not be overlooked.
Vibrio spp. microorganisms were detected in shrimp and ponds even when counts of fecal microorganisms were low. Thus, there may be no correlation between levels of fecal coliforms and the presence of Vibrio spp. Fecal coliforms have long been used as indicators of problematic bacterial contamination.
Shrimp Processing and Storage
A total of 1,264 samples of individually quick-frozen, peeled and deveined raw shrimp (pond-raised Penaeus monodon) and 914 samples of cooked, ready-to-eat shrimp were analyzed for their microbiological contents. The results are summarized in Table 1. Of the raw shrimp samples, 96 percent showed an aerobic plate count (APC) of less than 105 cfu/g, and 74 percent were less than 104 cfu/g. APC values of cooked, ready-to-eat shrimp were less than 104 cfu/g in 99 percent of the samples. Coliforms were found in 15 percent of raw shrimp samples but in only 3 percent of the cooked samples. Studies have shown that the total APC may increase even in adequately refrigerated shrimp, whereas the levels of coliforms and thermal-tolerant coliforms increase only when products are not stored at the correct temperature. Raw shrimp has been reported to contain coagulase-positive staphylococci, while cooked shrimp tested negative for this microorganism. Since the presence of staphylococci in raw shrimp is typically attributed to workers, the absence of Staphylococcus in cooked product indicates that no post-processing contamination has occurred.
Clostridium botulinum is a significant potential contaminant of seafood worldwide. Its incidence in fish and in outbreaks of food poisoning has been extensively studied. In recent years, the use of modified-atmosphere and high-barrier film packaging (MAP/VP) for seafood has increased; such packaging can create the anaerobic conditions C. botulinum requires for growth. Refrigeration is being used to extend the shelf life of fresh products at a reasonable cost, but some C. botulinum strains can grow at 3.3 °C.
Bacterial Content of Individually Quick-Frozen Raw and Cooked Ready-to-eat Shrimp.
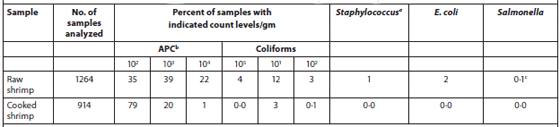
bAerobic plate count
cserotype Salmonella typhimurium
Aeromonads are another group of microorganisms ubiquitous in the aquatic environment. These bacteria have a broad host range and have often been isolated from humans with diarrhea. They are also primary pathogens in a wide range of cold-blooded animals. Environmental and clinical strains do not seem to differ with respect to the occurance of virulence factors.
Freshwater prawns have significant numbers of motile aeromonads that can grow at low temperatures, which sets the shelf life of iced freshwater prawns at 12 to 16 days. Pseudomonas, Aeromonas hydrophila, A. veronii, A. boivar, A. sobria and Shewanella putrefaciens are spoilage bacteria for fresh prawns. A study by Lalitha and Surendran (2006) reports that after 26 days of iced storage the APC of freshwater prawns exceeded 107 cfu/g, which is considered unsafe.
Oysters
Oysters are filter feeders that efficiently concentrate microorganisms found in the water and sediment. Because oysters are consumed raw, they pose a health risk to consumers. The consumption of raw oysters has been linked to outbreaks of acute gastroenteritis in both the Eastern and Western hemispheres.
Pathogenic Vibrio spp., specifically Vibrio vunificus, V. parahaemolyticus and V. cholerae non-01, are of concern in oysters. Vibrio spp. tend to be more abundant in warmer months than in colder months. Oysters harvested when the water temperature is below 76 °F (20 °C) usually contain lower numbers of any given Vibrio species. There are both clinical and environmental strains of Vibrio and one can not tell which is present unless someone becomes ill.
Nearly all Vibrio vulnificus strains found in oysters appear to be virulent, though genetic tests have not been able to distinguish fully virulent from less virulent strains, or environmental from clinical strains. In various studies, V. vulnificus was not detected in seawater, oysters or suspended particulate matter (SPM) samples during the cold winter months, but was detected at low levels in several sediment samples during this time period. Levels of the organism first increased in early spring in the sediment, then in SPM, and then in oysters. A major increase in V. vulnificus occurred only after the seawater temperature rose above 20 °C and the salinity was lowered below 16 ppt by the winter-spring rainfall. The highest V. vulnificus levels recorded were associated with SPM. These results suggest that V. vulnificus: 1) overwinters in a floc zone at the sediment-water interface, 2) is resuspended into the water column in early spring following changes in climatic conditions, 3) colonizes the surfaces of zooplankton that are also blooming during early spring, and 4) is ingested by oysters during normal feeding (Vanoy et al., 1992).
Cook et al. (2002) sampled 370 lots of oysters (Crassostrea virginica and Crassostrea gigas) in the shell at 275 different establishments (71 percent restaurants or oyster bars, 27 percent retail seafood markets, and 2 percent wholesale seafood markets) in coastal and inland markets throughout the United States over a 1-year period. The Vibrio vulnificus and Vibrio parahaemolyticus contents of the oysters were analyzed. The oysters were harvested from the Gulf of Mexico (49 percent), Pacific (14 percent), Mid-Atlantic (18 percent) and North Atlantic (11 percent) coasts and from Canada (8 percent). Tables 2 and 3 show the V. vulnificus and V. parahaemolyticus MPN (Most Probable Number) counts of the samples within each of eight density ranges. Densities of both bacteria in market oysters from all harvest regions followed a seasonal distribution, with the highest densities in the summer. The highest densities of both organisms, which often exceeded 10,000 MPN/g, were observed in oysters harvested from the Gulf Coast. The majority (78 percent) of lots harvested from the the North Atlantic, Pacific and Canadian coasts had V. vulnificus densities below the detectable level of 0.2 MPN/g; none exceeded 100 MPN/g. V. parahaemolyticus densities were greater than those of V. vulnificus in lots from the same areas, with some lots exceeding 1,000 MPN/g for V. parahaemolyticus. Overall, there was a significant correlation with salinity. Storage time significantly affected both V. vulnificus (10 percent decrease per day) and V. parahaemolyticus (7 percent decrease per day) densities in the market oysters.
Intertidal harvest is practiced extensively in some Pacific Northwest estuaries. This involves hand picking the shellfish after the tide recedes and placing them in large baskets. The baskets are left in the harvest area until the tide rises high enough for a vessel to retrieve and transport them to the processing plant. Intertidal harvest may expose oysters to favorable conditions for the growth of Vibrio and other bacteria, especially on sunny days. Nordstrom et al. (2004) determined that in oysters harvested this way the mean V. parahaemolyticus densities were generally four to eight times greater at maximum exposure (when the next high tide occurred) than at first exposure (when the initial low tide occurred). While pathogen counts were generally low (
Campylobacter is a common bacterial pathogen that has caused enteritis in humans worldwide. The genus Campylobacter comprises multiple species, of which the thermophilic Campylobacter jejuni, C. coli, C. lari and C. upsaliensis are the most important. Camplylobacter jejuni and C. coli account for the majority of enteric infections in humans. Campylobacter lari appears to be widely present in the environment but is rarely reported as a human pathogen.
The incidence of Salmonella infections has risen in recent years and many cases are linked to seafood (Centers for Disease Control and Prevention, 2000), particularly to shellfish. As harvesting areas become more populated, more human sewage (with accompanying pathogens) is discharged into coastal waters. In oysters harvested from 36 U.S. bays in the summer (12 each from the West, East and Gulf coasts) and 12 bays in the winter (four per coast), Salmonella was isolated from each coast and 7.4 percent of all oysters sampled tested positive for the bacterium. Isolates tended to be bay-specific, with some bays having a high prevalence of Salmonella while other bays had none. Oysters harvested in winter had much lower concentrations than those harvested in summer. The vast majority (78/101) of Salmonella isolates were Salmonella enterica serovar Newport, a major human pathogen, confirming the potential hazard of raw oyster consumption. Contrary to previous findings, no relationship was found between the presence of fecal coliforms in the water and Salmonella in oysters.
Abundance of Vibrio Vulnificus in Oysters at Retail; Data are Shown by Harvest State and Region.
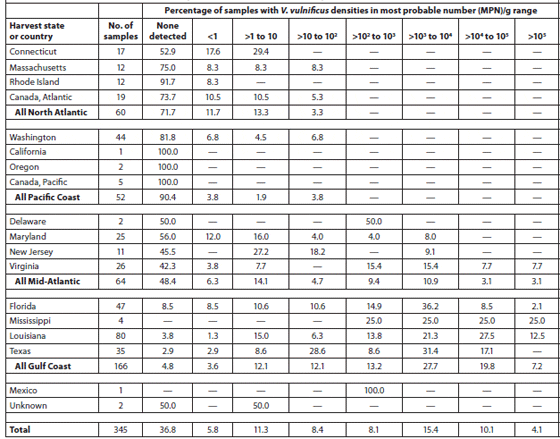
Aeromonads are ubiquitous throughout the environment and are found in both fresh and salt water. One member of the genus, Aeromonas hydrophila, is of particular concern and is believed to cause a form of foodassociated gastroenteritis. Aeromonas hydrophila has been reported to survive frozen temperatures of -72 °C for 1.5 years; it can grow and multiply at refrigeration temperatures.
Numerous viral illnesses have also been associated with the consumption of contaminated shellfish. Viruses likely to be transmitted by this route are enteric viruses, which can persist in the environment and can be concentrated by shellfish. In the 1960s, hepatitis A was the predominant viral disease resulting from the consumption of raw oysters, but now acute viral gastroenteritis has emerged as the most prevalent. However, hepatitis A is still a concern when raw oysters are consumed. In 2005, hepatitis A virus was confirmed as causing illness in four states among restaurant patrons who ate Gulf Coast oysters. Many cases of gastroenteritis are caused by small, round-structured viruses (SRSVs). An SRSV was implicated in an outbreak in oysters (Crassostrea virginica) harvested in Louisiana. Molecular characterization has shown that these viruses are human caliciviruses and that they are genetically related to the Norwalk virus.
Abundance of Vibrio Parahaemolyticus in Oysters at Retail; Data are Shown by Harvest State and Region.
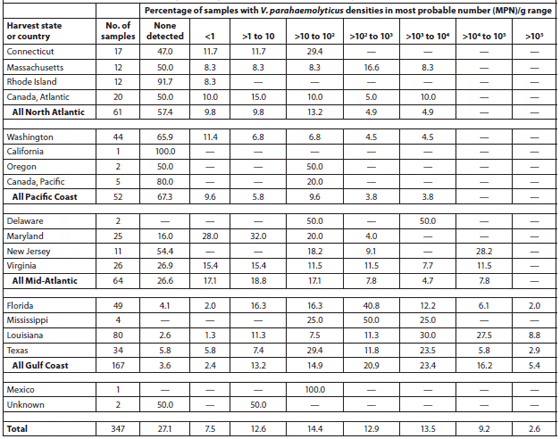
Post-harvest Processes for Oysters
Two treatments that reduce bacteria in fresh oysters are relaying and depuration.
Relaying. Relaying involves moving oysters harvested in a bacteriologically contaminated area (closed area) to an approved area (clean waters) for natural biological cleansing. Shellfish are collected under supervision and re-planted for at least one spawning season (June 15 to September 15). Specific relaying requirements vary from state to state, but National Shellfish Sanitation Program guidelines must be followed. Relaying has several benefits. It allows for the use of an otherwise unavailable resource; works over (cultivates) existing natural beds, which usually benefits the substrate; and further distributes a local gene pool, which may enhance natural spawning. The most significant benefit may be the depletion of natural stock from polluted areas, which reduces the incentive for illegal harvests. Illegal harvests of contaminated shellfish pose a significant public health hazard. Relaying is expensive, however, and careful oversight, testing and monitoring are required.
Depuration. Depuration is the purification of shellfish harvested from moderately contaminated areas under controlled conditions. The process generally involves holding shellfish in tanks of flowing seawater for 2 to 3 days. The seawater used is sterilized by ultraviolet light. Shellfish harvested from moderately contaminated areas normally purge themselves of bacterial contaminants within 48 hours, but they may be held for up to 72 hours in some circumstances. After that, quality and shelf life decline. Depuration is effective in removing many fecal bacterial contaminants from shellfish. As commercially practiced, it is less effective at removing viral contaminants such as norovirus and hepatitis A. It is not consistently effective in removing other contaminants such as naturally occurring marine vibrios (Vibrio parahaemolyticus and Vibrio vulnificus); marine biotoxins such as those causing paralytic shellfish poisoning (PSP), diarrheal shellfish poisoning (DSP), and amnesic shellfish poisoning (ASP); heavy metals; or organic chemicals.
The success of depuration and relaying depends on several factors, including shellfish metabolic activity as affected by water temperature, initial levels of pathogenic viruses or bacteria, water salinity, and specific design characteristics of the depuration system. Enteric viruses are very resistant to physical and chemical inactivation and may persist in shellfish tissues after depuration.
Processing Treatments of Oysters
High-pressure processing. Exposing certain foods to high pressure can reduce the microorganism count while retaining acceptable sensory properties. High-pressure treatment is very effective against Gram-negative organisms but less effective against Gram-positive ones. Vibrio parahaemolyticus in pure culture and in oysters was reduced to non-detectable levels when exposed to highpressure processing (HPP) at 345 MPa (mega pascals) for 30 and 90 seconds, respectively. Multiple strains of V. parahaemolyticus and V. vulnificus have been tested in phosphate-buffered saline to determine their sensitivity to HPP. The sensitivity among strains was variable in all species, with environmental species appearing more sensitive to HPP and clinical species tending to be more resistant. Kingsley et al. (2002) report that HPP (460 MPa for 5 minutes) also inactivates hepatitis A virus.
Gamma irradiation. Jakabi et al. (2003) studied the effect of gamma irradiation on both sensory attributes and high levels of Salmonella enteriditis, Salmonella infantis, and Vibrio parahaemolyticus in oysters (Crassostrea brasiliana). The oysters were exposed to gamma irradiation (60Co) in doses ranging from 0.5 to 3.0 kGy. A dose of 3.0 kGy was generally sufficient to reduce the level of Salmonella serotypes by 5 to 6 log10 units and did not change the odor, flavor or appearance of the oysters. A dose of 1.0 kGy was sufficient to produce a 6 log10 reduction in V. parahaemolyticus.
Electrolyzed oxidizing. The effect of electrolyzed oxidizing (EO) water treatment in reducing V. parahaemolyticus and V. vulnificus was investigated by Ren and Su (2006). Populations of V. parahaemolyticus (8.74 x 107 cfu/ ml) and V. vulnificus (8.69 x 107 cfu/ml) in pure culture decreased to nondetectable levels (
All Shellfish
Toxins
Many species of marine microalgae produce substances that are highly toxic. Because the algae are widespread and periodically form large concentrations in the form of population blooms, the amounts of toxins produced can be considerable. Mussels have been reported to accumulate substantial amounts of some phycotoxins faster than other bivalves (Shumway, 1990). Although mussels may acquire phycotoxins by ingesting bacteria or dissolved toxins, the most important route of acquisition is the ingestion of toxic phytoplankton. There is substantial variability in the uptake rate among individuals of the same species. Mussels are highly resistant to saxitoxin and similar compounds and are usually unaffected by feeding on dinoflagellates that produce the toxin (which causes paralytic shellfish poisoning, PSP). This resistance, along with the high filtration rate of mussels, is likely the reason mussels accumulate phycotoxin more quickly than other bivalves. Smaller mussels tend to have a greater concentration of toxins per unit weight than larger mussels.
Parasites
Among the protozoan parasites of animal and human interest, Cryptosporidium is very important for its threat to public health. Cryptosporidium is present worldwide and causes diarrheal disease in humans, which can be life threatening to immunocompromised individuals. Many factors facilitate Cryptosporidium accumulation in bivalues and transmission to humans: 1) the small size of the oocysts, 2) their viability in the environment (up to 1 year in artificial seawater), 3) the high concentrations reported in the feces of young calves (108 oocysts per g), 4) their low sedimentation rate, 5) their resistance to commonly used disinfectants, 6) the low doses required for human infection (< 30 oocysts), and 7) the lack of specific treatment.
The presence of Cryptosporidium oocysts in mussels has been reported in samples from the U.S., Europe and Canada. In one study, oocysts incubated in artificial seawater at 6 to 8 °C under moderate oxygenation were found to remain infectious for 1 year. This suggests that C. parvum can survive in seawater for at least 1 year and that seawater and mussels are a potential source of C. parvum infection for humans.
Cryptosporidium parvum has been detected in eastern oysters (Crassostrea virginica) at all commercial harvesting sites tested in Maryland tributaries to the Chesapeake Bay. It has also been found in oysters from 64.9 percent of sites sampled in 13 Atlantic Coast states from Maine to Florida and in New Brunswick, Canada (Fayer et al., 2003).
Customary processing operations may not completely eliminate C. parvum oocysts from shellfish. The parasite can survive in the tissue of fresh shellfish from harvest through consumption and is resistant to treatment with many toxic chemicals (both liquids and gasses). It also can survive several months at refrigeration temperatures. Some post-harvest methods used to destroy the oocysts include: 1) steaming at atmospheric pressure until all mollusks open their valves, 2) E-beam irradiation (1.0, 1.5 and 2.0 kGy), 3) microwave energy, and 4) irradiation exposure of 1 kGy. Unfortunately, none of these processes has been completely effective. High-pressure processing using 550 MPa for 180 seconds produced the maximum decrease (93.3 percent) of mouse infectivity from the oocysts.
Conclusions
Oysters and mussels can cause food-borne illness in both normal and immunocompromised individuals. Research has shown that various indicator microorganisms (such as fecal coliforms) do not accurately identify the risk of consuming raw or minimally cooked products. The best method of controlling pathogens is to use a postharvest treatment. Some treatments, such as pasteurization, irradiation, high hydrostatic pressure processing, and long-term freezing, reduce both pathogenic microorganisms (viruses, parasites) and the allergenic nature of specific proteins in shellfish species. However, not all processes are effective on all microorganisms. Some traditional processes, such as depuration, remove some pathogenic organisms but not all of them. Depuration is effective only when the shellfish are placed in clean waters for extended time periods. The time required will depend on the shellfish species, the amount of pathogen accumulation, the type of pathogen, and environmental factors.
Some shellfish, such as shrimp, are usually eaten fully cooked. The major health hazards with these products are contamination during or after processing and allergenic reaction. Shrimp are also hazardous if they scratch or wound handlers because such injuries can cause infection.