Aquaculture continues to expand, with a gradual shift from traditional, low-cost semi-intensive systems to more costly intensive systems, where processed feed is a major component. As a result, total industrial processed aquafeed production has increased from 7.6 million tonnes in 1995 to 35 million tonnes in 2010, with an average annual growth rate of about 11 per cent. It is also expected that this growth rate will continue, and aquafeed production will reach 70 million tonnes by 2020 (Tacon 2012; Tacon et al. 2012). The aquaculture feed sector consumed over 73 per cent of the total global fishmeal production and over 80 per cent of the total global fish oil production in 2010 (Tacon 2012).
Fishmeals, which are the primary protein source in aquafeed industry, have become costly and of limited availability (Tacon et al. 2012). In the meantime, plant-based protein sources, particularly oil plant sources, have been receiving considerable attention over the past few decades as a partial or total fishmeal replacer in aquafeed industry (Tacon et al. 2011). However, most ingredients of plant origin are limited in a number of nutrients, including taurine, which might be necessary for the optimal performance and metabolism of farmed aquatic animals, particularly for carnivorous fish.
The beneficial effects of taurine on mammals have been extensively investigated for several decades (Huxtable 1992; Stapleton & Bloomfield 1993; Di Leo et al. 2002; Lourenco & Camilo 2002; Kuzmina et al. 2010). However, only recently, the effects of taurine on fish physiology, metabolism, culture and nutrition have been increasingly researched in different freshwater and marine fish species.
A good number of publications appeared on this subject during the past two decades, with varying results. It is my belief that comprehensive review and critical analysis of the role of taurine in aquaculture, and nutrition in particular, appear timely. This review will tackle this issue, through analysing and discussing the available studies that have been conducted on synthesis and functions of taurine in fish and its use in aquaculture and aquafeeds. It is hoped that this review will improve our understanding of taurine and its role in aquaculture.
Taurine structure and functions
Taurine, or 2-aminoethanesulfonic acid, is an end product of metabolism of sulphur-containing amino acids. It is a neutral β-amino acid, where both the amine group and sulphonic group can be ionized (Jacobsen & Smith 1968). Taurine is not incorporated into proteins, nor degraded by mammalian tissues (Kuzmina et al. 2010). However, it is the most abundant free amino acid in animal tissue, because it accounts for 30–50 per cent of the entire amino acid pool, depending on the animal species (Jacobsen & Smith 1968). Taurine is concentrated mainly in the heart, retina, skeletal muscle, brain, large intestines, plasma, blood cells and leucocytes in mammalian tissues (Huxtable 1992; Schuller-Levis & Park 2003).
In mammals, taurine is involved in many biological functions, including antioxidation and detoxification (Stapleton & Bloomfield 1993), modulation of immune response, calcium transport (Takahashi et al. 1992), myocardial contractility, retina development (Omura & Yoshimura 1999), bile acid metabolism (Hofmann & Small 1967), osmotic regulation (Thurston et al. 1980) and endocrine functions (Huxtable 1992; Di Leo et al. 2002; Lourenco & Camilo 2002; Kuzmina et al. 2010). This amino acid also plays a significant role in reducing the loss of potassium from heart muscles, and therefore can prevent the onset of hazardous cardiac arrhythmias and related heart disorders in humans (Eby & Halcomb 2006). Taurine has hypoglycaemic and antioxidant properties, suppresses progressing of vascular complications in humans and preserves secretory ability of pancreatic cells (Hansen 2001). It also increases sensitivity of tissue receptors to insulin (Hansen 2001; Franconi et al. 2004). Taurine is also essential in the regulation and control of serum cholesterol levels in the blood in humans (Lourenco & Camilo 2002).
Taurine biosynthesis and metabolism
Taurine biosynthesis is one of the most important catabolic processes of sulphur-containing amino acids in most mammalian species (Kuzmina et al. 2010). The predominant route of taurine synthesis varies between species and depends on the type of tissue. For example, the rate of taurine synthesis in rats is higher than in human, whereas cats lack the ability to synthesize this amino acid due to a limited activity of cysteinsulphinate decarboxylase (CSD) (Knopf et al. 1978; Hayes & Trautwein 1989). CSD decarboxylates cysteinesulphinate to hypotaurine, which, in turn, is oxidized to taurine (Griffith 1987). Endogenous taurine synthesis occurs mainly in the liver and brain in many mammalian species through enzymatic oxidation and direct conversion of cysteine, or conversion of methionine into cysteine (Jacobsen & Smith 1968; Stapleton et al. 1997; Stipanuk 2004; Chang et al. 2013). In rat liver, ‘taurine pathway’ accounts for 70 per cent of degradation of cysteine to pyruvate or taurine (Yamaguchi et al. 1973).
In mammals, this process involves the actions of cysteine dioxygenase (CDO), which leads to cysteinesulphinate, and CSD (Griffith 1987). Pyridoxine (vitamin B6) is also required to convert cysteine or methionine to taurine (Brosnan & Brosnan 2006).
Historically, taurine has not been considered as an essential nutrient for fish (Robinson et al. 1978; Borlongan & Coloso 1993; Yokoyama & Nakazoe 1996; Akiyama et al. 1997). Just recently, studies have indicated that taurine synthesis widely differs between fish species, depending on fish species and size, feeding habits and cysteinesulphinate decarboxylase (CSD) activity.
Marine fish species, such as Japanese flounder (Paralichthys olivaceus), red sea bream (Pagrus major) and yellowtail (Seriola quinqueradiata), have low or negligible ability of taurine synthesis due to the absence of or low CSD activities during intermediate metabolism from methionine to cystathionine (Goto et al. 2001a; Yokoyama et al. 2001; Park et al. 2002; Kim et al. 2003, 2005a, 2008a; Takagi et al. 2005, 2006a,b, 2008, 2011). Therefore, supplemental taurine may be indispensible, particularly if they are fed plant-based food. On the other hand, freshwater teleost fish, such as rainbow trout, Atlantic salmon and common carp can synthesize taurine through transsulphuration pathway (Goto et al. 2001a; Yokoyama et al. 2001; Espe et al. 2008, 2012; Kim et al. 2008a).
Tissue taurine concentrations of these fish increased by dietary methionine and cystine supplementation (Cowey et al. 1992; Yokoyama & Nakazoe 1992; Espe et al. 2008). Bluegill (Lepomis macrochirus) (Goto et al. 2001a, 2004) also have the ability to synthesize taurine, despite that the activity of CSD in bluegill fed a taurinedeficient diet was significantly lower than that of fish fed a high taurine diet (FM) (Goto et al. 2004). It appears from these results that the natural feeding habits and feeding histories of the fish may affect taurine biosynthesis, through the effects on the activity of CSD (Gaylord et al. 2006). This may explain the ability of carnivorous fish (such as Atlantic salmon, rainbow trout and channel catfish) and the inability (or low ability) of herbivorous fish (such as grass carp and tilapia) to synthesize taurine from methionine or cysteine.
More discussion on the relationship between taurine synthesis/requirement and feeding habits of the fish is provided later, under the heading ‘Effects of taurine on the performance of farmed fish and shrimp’. A full section ‘The relationship between fish size, feeding habits and taurine requirement’ has been allocated to describing this relationship.
Physiological functions of taurine in fish
As in mammals, taurine is involved in many physiological functions in fish, including osmotic regulation, antioxidation, feeding stimulation and retina development and vision. This section briefly highlights the published works on these functions in different fish, crustacean and molluscan species.
Because taurine comprises 30–>50 per cent of the free amino acid pool in blood, muscles and brains of animals, including fish (Jacobsen & Smith 1968; Saha et al. 2000, 2002), it may play a significant osmoregulatory role in the cellular and central nervous system of marine fish species such as flounder (Pseudopleuronectes americanus) (King et al. 1982) and skate (Raja erinacea) (Ballatori & Boyer 1992) as well as freshwater fish such as walking catfish (Clarias batrachus) (Saha et al. 2000), tilapia and carps (Takeuchi et al. 2000, 2001a). For example, when walking catfish levers were perfused with isotonic medium, their taurine concentrations were lower than in fresh levers (Saha et al. 2000, 2002). The high physiological hepatic taurine concentration by the perfused liver suggests that taurine plays an important role in cell volume homoeostasis in walking catfish (Goswami & Saha 2006).
Similar findings have been reported in skate (Raja erinacea) (Ballatori & Boyer 1992) and trout (Oncorhynchus mykiss) (Michel et al. 1994). Takeuchi et al. (2000, 2001a) also found significant increase in taurine transporter mRNA in carp and tilapia tissues during salinity-induced stress. The involvement of taurine in osmoregulation of crustacean and molluscan species has also been reported by many authors. Dalla Via (1986, 1989) found that the most abundant individual FAAs engaged in ionic regulation of juvenile penaeid shrimp (Penaeus japonicas) and prawn (Palaemon elegans) were glycine, taurine, arginine, proline and alanine. Similar results have been reported in freshwater prawn (Macrobrachiumrosenbergii) (Tan & Chong 1981), where their transfer from diluted to more concentrated media caused a significant increase in FAA concentration in muscle tissue and a decrease in haemolymph protein content. Taurine has also been reported to accumulate in intracellular spaces of aquatic molluscs and is used as an osmolyte (Hosoi et al. 2008).
Taurine is also known to have antioxidant, membranestabilizing properties, as it inhibits lipid peroxidation, and thus prevents cell death in animals and humans (Patrick 2006). Antioxidative and protective effects of taurine in fish have recently attracted the attention of a number of authors. Rosemberg et al. (2010) found that taurine treatment resulted in a significant reduction in lipid peroxides in zebrafish exposed to ethanol, due to the increase in total reduced thiol content detected after taurine pretreatment and alcohol exposure. Taurine has also been shown to alleviate cadmium (Cd)-induced oxidative stress in freshwater catfish (C. batrachus) (Kumar et al. 2009). Similarly, taurine was able to counteract oxidative stress induced by exposure to Cd in common carp (Cyprinus carpio) (Sevgiler et al. 2011). Taurine can also protect body cells against oxidation, by protecting mitochondrial integrity and respiration, as has been demonstrated in Atlantic salmon exposed to CdCl2 (Espe & Holen 2013).
Bile acids are steroids derived from cholesterol, synthesized by the liver, stored in the gall bladder and released into the intestinal lumen to emulsify fats and help in the absorption of lipids and fat-soluble vitamins (Haslewood 1967).
Bile acids are conjugated mainly with taurine, and— to a lesser extent—with glycine. Therefore, taurine may enhance lipid metabolism in fish, through the increase in the activity of the bile salt-activated lipase in the liver, as has been reported in common dentex (Dentex dentex) (Chatzifotis et al. 2008). Bile salts (or free taurine) can also be used as olfactory stimuli for fish, and in turn, increase feed consumption and/or change feeding behaviour (Sola & Tosi 1993; Chatzifotis et al. 2009).
Marine fish such as European glass eel Anguilla anguilla (Sola & Tosi 1993), European sea bass Dicentrarchus labrax fry (Brotons-Martinez et al. 2004) and gilthead sea bream Sparus aurata fry (Chatzifotis et al. 2009) responded to taurine-supplemented feeds more actively than taurine-free or taurinelow feeds. Similar responses to dietary taurine have also been reported in some freshwater fish. Both taurine- and glycine-conjugated bile acids were more stimulatory in rainbow trout (Yamashita et al. 2006) and channel catfish Ictalurus punctatus (Rolen & Caprio 2008) gustatory systems. Feeding behaviour of juvenile Japanese flounder was also affected by dietary taurine (Kim et al. 2005b). Taurine supplementation (0.5 and 1.5 per cent) led to the immersion of the fish onto the bottom immediately after feeding, whereas the fish were swimming in the water column in the absence of taurine.
The effects of dietary taurine levels and lipid contents on the conjugated bile acid composition of juvenile Japanese flounder were further investigated by Kim et al. (2008b). When fish juveniles (0.04 g) were fed diets containing increasing concentrations of taurine (0.5–1.5 per cent) at two lipid levels (0 and 5 per cent), body taurine contents increased with increasing dietary taurine. The conjugated bile acids in the gall bladder also increased with the increase in the dietary taurine level and consisted of taurocholic acid (which accounted for more than 95 per cent of the total conjugated bile acids) and taurochenodeoxycholic acid. These results suggested that taurine is the sole precursor of conjugate bile acid in Japanese flounder juveniles.
Taurine supplementation plays a significant role in preventing green liver syndrome. This effect has been attributed to the increase in the excretion of bile pigments from the liver into the bile and the decrease in the production of haemolytic biliverdin (Takagi et al. 2005, 2006a). The effects of dietary taurine in the prevention of green liver syndrome have been demonstrated in red sea bream (Goto et al. 2001b; Takagi et al. 2006b, 2010, 2011). When yearling fish were fed high SPC diets without taurine supplementation, they exhibited inferior growth rates and feed efficiency, together with higher incidence of green liver (Takagi et al. 2006b, 2010). The hepatopancreatic taurine concentration was also significantly lower, and hepatopancreatic biliverdin concentration was higher compared with the fish fed a FM-based diet. Taurine supplementation improved fish performance and alleviated these symptoms.
Taurine is an important neurochemical factor in the animal visual system (Omura & Yoshimura 1999; Militante & Lombardini 2002). Visual dysfunction in both human and animals may result from taurine deficiency (Militante & Lombardini 2002). This deficiency can be reversed with exogenous taurine supplementation. A number of studies have been carried out on the role of taurine on retinal development and visual system in fish. It has been reported that abundant taurine is localized in the retinal photoreceptor and neural layers of juvenile flounder (Omura & Yoshimura 1999) and goldfish (Nusetti et al. 2009). These results suggest that taurine may be involved in the protection of the photoreceptor outer segment, the regulation of neural transmission and photoreceptor differentiation during the developmental stages. In addition, Nusetti et al. (2009) found that taurine transporter, taurine and zinc also coexist in photoreceptors and the ganglion cell layer, suggesting that both taurine and zinc are important in normal cellular functions in fish retina.
Dietary sources of taurine
Animal protein sources, such as meat, sea foods (fish, oysters, mussels, clams, shrimp, crabs, etc.), eggs and dairy products, contain high levels of taurine (Jacobsen & Smith 1968; Spitze et al. 2003). Marine algae also contain varying levels of taurine. On the other hand, higher plant tissues (such as soybean) are generally deficient in taurine. Taurine contents of the common food sources and feedstuffs are summarized in Table 1.
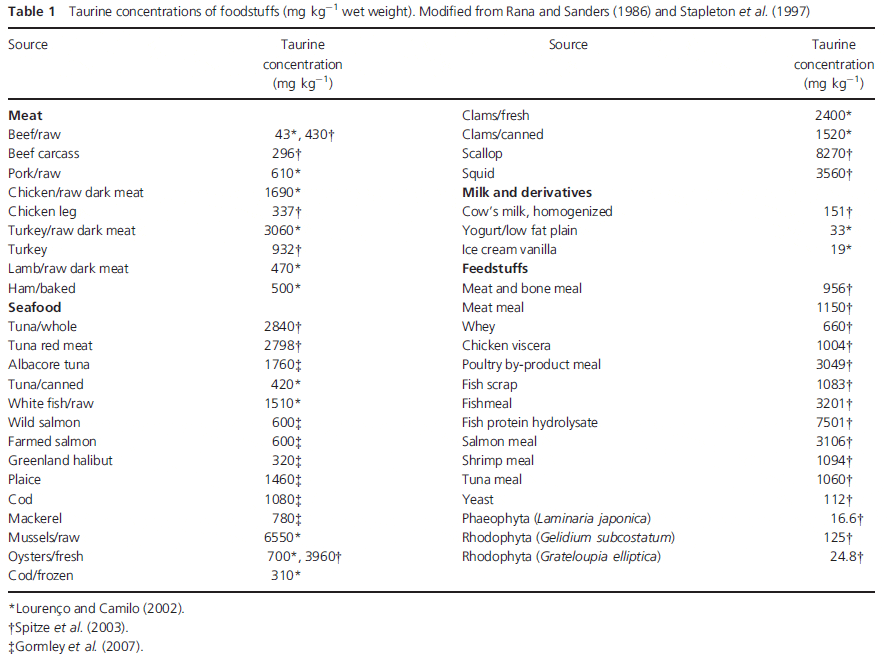
Effects of taurine on the performance of farmed fish and shrimp
In recent years, several publications appeared on the beneficial effects of supplemental dietary taurine on the growth, survival, feeding behaviour, feed consumption and feed utilization efficiency of different fish species and sizes. This section reviews and analyses these studies. For facilitating the issue, and assisting the reader extract the necessary information, the major results were piled in a comprehensive table (Table 2) (Please click on link below).
Freshwater fish
As mentioned previously, exogenous taurine may not be essential for many freshwater fish species, because they have the ability to synthesize it. Studies on taurine supplementation in farmed fish feeds indicated that supplemental methionine and cystine with dietary taurine or inorganic sulphate have proved unsuccessful with fingerling channel catfish (Robinson et al. 1978) and rainbow trout (Page 1978). When these fish were fed dietary taurine, they exhibited reduced growth and development of cataractogenesis in rainbow trout (Page 1978).
Similarly, taurine was not beneficial for fingerling and juvenile rainbow trout when they were fed a methionine- or hydroxy analogue (MHA)-supplemented feed (Yokoyama & Nakazoe 1992; Boonyoung et al. 2012). When these fish were fed soy protein concentrate (SPC) diet supplemented with MHA, they exhibited higher plasma taurine levels, presumably due to the conversion of dietary MHA into taurine, leading to promoted fish growth (Boonyoung et al. 2012).
These results suggest that dietary MHA may spare taurine in terms of promoting the performance of rainbow trout, while taurine had no effects on fish fed both the SPC- and MHA-based diets. The final levels of taurine in the fish and fillet of rainbow trout juveniles fed plant proteins (full fat soy, extracted soy, soy protein concentrate, corn gluten) which are deficient in taurine, and fish hydrolosate (rich in taurine) were almost similar, regardless of dietary taurine levels (Aksnes et al. 2006). Therefore, taurine seems to be homoeostatic regulated in these fish independently of dietary taurine levels. This particular finding suggests that plant protein sources may be included in diets for trout at high levels without affecting the feeding quality as evaluated by taurine levels.
Studies on other freshwater species including Atlantic salmon (Salmo salar) juveniles (Espe et al. 2012) and common carp fry (Fontagne et al. 2000; Carvalho et al. 2004) and juveniles (Kim et al. 2008a) indicated also that dietary taurine supplementation is not necessary for optimum performance and survival. These fish are able to synthesize taurine from methionine and cysteine through transsulphuration pathway (Espe et al. 2008, 2012). Similar results were also reported in juvenile red drum (Sciaenops ocellatus) (Patterson et al. 2012) reared in brackish water (7 per cent). The nonessentiality of dietary taurine for these freshwater fish has been attributed to the transsulphuration of dietary methionine, leading to an increase in body taurine in fish fed taurine-free (or taurine-deficient) diets (Espe et al. 2008). The biosynthesis of taurine from methionine or cysteine has also been reported in adult rainbow trout (Yokoyama & Nakazoe 1991; Yokoyama et al. 2001). Taurine was accumulated in the whole body, liver and muscle when rainbow trout were fed with dietary sulphur amino acids, supporting the ability of taurine biosynthesis in these fish. Subsequently, Yokoyama et al. (2001) reported that the activity of cysteinesulphinate decarboxylase (CSD) was high in rainbow trout.
In contrast to the above findings, a number of recent studies indicated that freshwater fish may require exogenous taurine for optimum growth performance, feed consumption, digestion and assimilation and other physiological functions. This requirement depends on fish species, size and feed composition. For example, Gaylord et al. (2006, 2007) reported that taurine supplementation (0.5 per cent) was essential for optimal performance of juvenile rainbow trout fed SPC-based diets (deficient in methionine), while no effects of dietary taurine were observed in fish fed FM-based diets (rich in methionine and taurine). Similarly, taurine supplementation improved growth rates, feed digestibility, feed efficiency, hepatopancreatic and intestinal protease, lipase and amylase activities of juvenile carp (species not provided) (Liu et al. 2006; Chunsheng et al. 2007), grass carp (Ctenopharymgodon idellus) (Li et al. 2005; Luo et al. 2006) and Nile tilapia (Oreochromis niloticus) larvae (Goncalves et al. 2011) fed plant protein– based diets. It is evident from these studies that taurine is conditionally essential when these fish are fed diets containing high plant protein levels and deficient in methionine and/or cysteine. The essentiality of taurine for freshwater fish may also be affected by the feeding habits and previous feeding histories of these fish, as will be discussed later.
Marine fish and shrimp
Much more efforts have been directed to the effects of taurine on cultured marine fish than freshwater fish. However, most of the studies have been focusing on only few species, namely Japanese flounder (Paralichthys olivaceus), sparid fish (red sea bream Pagrus major and common dentex (Dentex dentex), yellow tail (Seriola quinqueradiata), turbot (Scophthalmus maximus), European sea bass (Dicentrarchus labrax) and cobia (Rachycentron canadum), in addition to a few studies on marine shrimp. These studies indicated that taurine may be conditionally essential for these fish species. The effects of supplemental taurine on the performances of these species depend on a number of factors including dietary protein sources, fish species and size, natural feeding habits and previous feeding history of the fish.
The relationship between dietary protein source and taurine requirement
As mentioned earlier, plant protein sources (such as SBM) are deficient in taurine or its precursors, namely cysteine and methionine (Espe et al. 2008). The inclusion of high levels of plant protein in marine fish feeds is expected to cause deterioration in fish performance, possibly due to the inability, or low ability, of these fish to synthesize taurine. For example, when red sea bream (P. major) were fed on high plant protein diets (SBM, SPC), they were unable to biosynthesize taurine from cystine (Goto et al. 2001b; Takagi et al. 2006b, 2011; Matsunari et al. 2008a). Taurine supplementation (0.5–1 per cent) was necessary for optimum growth rates, feed efficiency, body taurine and physiological conditions. Supplemental taurine (0.2 per cent) also improved growth rates, feed efficiency and lipid metabolism of common dentex (Dentex dentex) juveniles fed SPC-based diets (Chatzifotis et al. 2008). The effects of taurine supplementation on growth performance of European sea bass (Dicentrarchus labrax) larvae (Brotons-Martinez et al. 2004) and juveniles (Kotzamanis et al. 2012) fed on SBM-based diets have been evaluated. Feeding activity and growth rates were improved with increasing dietary taurine supplementation. These results suggested that sea bass fry require 0.2 per cent dietary taurine, while juvenile fish required 1 per cent dietary taurine for optimum performance.
Several trials have also been carried out to reduce the FM content in the diets of yellowtail, which is highly carnivorous, by increasing the inclusion of plant protein sources (mainly soybean protein) in these diets. These diets have generally led to inferior performance and deterioration in some physiological functions, mainly due to the deficiency of taurine (and other amino acids) in these plant sources, compared with fishmeals (Watanabe 2002; Takagi et al. 2008; Nguyen et al. 2011a,b). Taurine synthesis has been reported to be extremely low or even negligible in yellowtail (Takagi et al. 2005). The activity of CSD during intermediate metabolism from methionine to cystathionine is absent or markedly low in these fish (Matsunari et al. 2005a). Thus, it is expected that dietary taurine supplementation would be necessary for optimum performance and physiological functions of these fish when they are fed on plant protein–based diets.
In support, early stage yellowtail juveniles (0.5 g) fed on FM-based diet required only 1 per cent taurine for optimum growth performance (Matsunari et al. 2005a), while larger juveniles (470 g) fed SPC-based diets required much higher dietary taurine (up to 4.5 per cent) (Takagi et al. 2006a, 2008). In addition, Takagi et al. (2005) reported that growth of juvenile yellowtail fed with SBM-based diets (58 per cent soybean protein) was improved by 3 per cent taurine supplementation.
Fish fed the SBM diet without taurine supplementation resulted in inferior growth rates, low feed efficiency, high mortality and anaemia, concomitant with higher incidence of green liver. This suggested that plant protein–based diets increase the requirement for taurine in yellowtail juveniles, possibly to increase synthesis of taurine- conjugated bile acids and to compensate for its excretion from the intestine (Nguyen et al. 2011a,b).
It is clear from this discussion that taurine is conditionally essential for the investigated marine fish, when they are fed diets containing high concentrations of plant protein sources (such as SBM) or diets deficient in sulphur amino acids. In the meantime, Lunger et al. (2007) found that when juvenile cobia (Rachycentron canadum) fed diets containing 50 and 75 per cent yeast-based protein supplemented with taurine alone, taurine, methionine and tryptophan or methionine and tryptophan alone, the best performance was recorded in fish fed the yeast diets supplemented with all three amino acids followed by the diets supplemented with taurine alone. The authors concluded that when taurine was supplemented alone, it allowed cobia to conserve the essential amino acids (methionine and tryptophan), leading to improving growth rates.
Processing of plant protein sources and taurine supplementation may also improve fish performance, feed digestion and synthesis of taurine-conjugated bile acids in yellowtail (Nguyen et al. 2011a,b). Nguyen et al. (2011a) investigated the effects of SBM fermentation and taurine supplementation on lipid digestion and growth of fingerling yellowtail fed SBM-based diets. Supplemental taurine or both taurine and fermentations improved lipid digestibility, lipase activity, lipid content and bile acid concentration. However, growth rates were improved only by SBM fermentation with taurine supplementation.
Similarly, growth rates, body taurine and bile acid levels of juvenile yellowtail fed diet containing digested and purified soy protein isolate (DPSPI) as protein sources were better than that of fish fed undigested, high molecular fraction (HMF) of soybean protein, soybean meal (SBM), soy protein isolate (SPI), digested SPI (DSPI) (Nguyen et al. 2011b). In addition, taurine (1 per cent), cholesterol (1 per cent) and their combination (1:1) resulted in a better growth rate of juvenile turbot fed high SBM diets than the control diet (Yun et al. 2012). Fish fed the taurine diet exhibited the highest activity of cholesterol 7α hydroxylase, followed by those fed the cholesterol and the control diet, respectively.
The relationship between fish size, feeding habits and taurine requirement
In addition to the effects of protein source on taurine synthesis, fish size has also been reported to affect taurine synthesis and metabolism in marine fish species. Extensive research has recently been carried out on the effects of exogenous dietary taurine supplementation on Japanese flounder, at different growth stages, in terms of growth rates (Park et al. 2002; Kim et al. 2005a,b), feeding behaviour (Kim et al. 2005b), conjugated bile acids contents (Kim et al. 2007, 2008b), sulphur amino acid metabolism (Park et al. 2002; Kim et al. 2003) and taurine biosynthesis (Kim et al. 2005a, 2008a). These studies indicated that Japanese flounder are unable to biosynthesize taurine from cystine, particularly during early stage juveniles (0.04– 0.4 g).
Supplemental taurine (1.5–2.0 per cent) was necessary for the improvement of growth, feeding behaviour, body taurine and sulphur amino acid metabolism, even if the fish were fed with a taurine-rich protein sources (combined mix of fish, krill and squid meal) (Park et al. 2002; Kim et al. (2005a). This has been attributed the dependence of early stage flounder after settlement on taurine-rich mysids as the main natural food source (Hirota et al. 1990; Tanaka 1993; Furuta 1996; Sekai et al. 1997). On the other hand, larger fingerlings (15 g) were not affected by taurine supplementation (Kim et al. 2003). The growth rates and body free taurine of turbot (S. maximus) larvae fed on natural zooplankton or Artemia were also positively correlated with dietary taurine concentration, suggesting a dietary dependence of these larvae on taurine and/or sulphur amino acids (Conceicao et al. 1997; Yun et al. 2012).
In addition, small juvenile turbot (6.3 g) fed casein-based diets required higher dietary taurine (1.0 per cent) for optimum performance, whereas larger fish (165.9 g) required only 0.5 per cent taurine (Qi et al. 2012). Matsunari et al. (2005a) found also that supplementation of taurine (1 per cent) to a FM-based diet fed to early stage yellowtail juveniles (0.5 g) for 6 weeks improved their growth performance over the first 3 weeks. Afterwards, growth performance was not significantly related to taurine supplementation.
Taurine supplementation has also been reported to improve not only growth rates, but also feed consumption and feed utilization of small fish sizes. Qi et al. (2012) found that both feed intake and feed utilization and growth rates increased in small turbot (6.3 g) by taurine supplementation. The authors suggested that increased growth was probably due to the increase in feed intake and feed utilization. In larger turbot (165.9 g), feed intake was significantly increased by supplementation of taurine, but feed utilization was not significantly enhanced. This suggests that the increased growth may have been attributed to the increase in feed intake, but not feed utilization efficiency. Similar results have been reported in red sea bream (Matsunari et al. 2008b; Takagi et al. 2011).
It is evident from these studies that the response of marine fish to dietary taurine is affected by fish size (body weight). This phenomenon has been attributed to the taurine polyfunctionality appearing at different life stages of the animal (Kuzmina et al. 2010). The developmental stage, natural feeding habits and previous feeding histories of marine fish may therefore have a significant impact on taurine requirement and synthesis through the effects on the activity of CSD (Gaylord et al. 2006). This supports the assumption that taurine may be a conditionally essential amino acid for larval and early juvenile stages of marine fish such as Japanese flounder, turbot and yellowtail, which feed on taurine-rich foods during their early developmental stages. However, Kim et al. (2007) reported that taurine supplementation improved the growth and feed efficiency of both juveniles (0.7 g) and larger fingerlings (9.6) of Japanese flounder. The authors related this difference between their results and the previous studies (Kim et al. 2003) to the dietary history of the fish before the start of the study. This particular result suggested that dietary taurine might be an essential nutrient in both early and later life stages of Japanese flounder.
Supplemental taurine has also been reported to improve reproductive performance of yellowtail broodstock (Matsunari et al. 2006). The fish were fed diets containing 40 per cent FM and 24 per cent SBM and supplemented with 0.0, 0.5 and 1.0 per cent taurine for 5 months prior to spawning. Oocyte growth improved significantly with the increase in dietary taurine. Spawning success for females at these taurine concentrations was 0.0, 14 and 86 per cent, respectively. Fertilization rate was also significantly increased, while eggs abnormality decreased, with increasing dietary taurine. The taurine concentrations in the liver and serum also increased with increasing taurine supplementation. This result suggested that supplemental taurine is an essential nutrient for broodstock yellowtail, as it was found essential for larval and juvenile marine finfish, such as Japanese flounder and red sea bream (Takeuchi et al. 2001b).
Marine shrimp
Few trials have considered the effects of supplemental taurine on physiological functions and growth performance of shrimps. These studies indicated that shrimp requires this amino acid for optimum performance during both larval and grow-out stages. Shi-Yen and Ben-Shan (1994) found that grass shrimp (tiger shrimp) Penaeus monodon fed purified diets supplemented with 0.4–0.8 per cent taurine had significantly higher growth and utilization efficiency and serum cholesterol concentrations than at lower taurine concentrations (0.0 and 0.2 per cent). White shrimp (Litopenaeus vannamei) fed low-fishmeal diets supplemented with taurine (0.04–0.4 per cent) required 0.17 per cent taurine for maximum growth rates (Yue et al. 2012). Supplemental taurine (10 and 25 mg kg -1) also resulted in speeding up moulting rate of white shrimp larvae and significantly improved survival rate (Mayasari 2005). At higher taurine concentrations (50 and 100 mg kg -1), all the larvae died, possibly due to the poisonous effect of taurine when provided at excessive levels. However, further research is needed to support this assumption.
Richard et al. (2011) evaluated the sparing effect of choline and cystine on methionine requirements for protein accretion in P. monodon juvenile (33 g) by measuring the activity of two key enzymes of remethylation (betaine– homocysteine methyltransferase; BHMT) and transsulphuration (cystathionine b-synthase; CBS). They found that P. monodon has the ability to synthesize taurine, and this ability is significantly affected by the cystine level in the 30 per cent SAA-limiting diets. The authors suggested that further research is needed to better understanding of the sparing effects of both choline and cystine on methionine requirements and also for characterizing the pathways regulating taurine synthesis in this species.
Live food enrichment with taurine
It has been reported that rotifers Brachionus plicatilis and B. rotundiformis, which are indispensable live food organisms for marine fish larvae, are deficient in taurine (Takahashi et al. 2005). Enriching rotifers with taurine may be necessary for improving larval rearing of marine fish. Therefore, enrichment of live food with exogenous taurine for marine fish larvae has been extensively investigated and revealed that taurine is essential for larval development and growth. Takahashi et al. (2005) found that the content of taurine in the enriched rotifers increased with increasing taurine in the medium and becomes constant after 16 h. The authors found also that enriched rotifers contained about 75 per cent of initial accumulated taurine after 8 h from final enrichment. Salze et al. (2011) found that live rotifers and artemia enriched with 4 g L-1 per day of taurine benefited larval morphology, development (ontogeny of the lateral line system, olfactory organ and gills), growth and survival of cobia R. canadum. The same authors (Salze et al. 2012) found that cobia larvae fed either bioencapsulated diets or rotifer and artemia nauplii enriched with 4 g taurine L -1 per day exhibited improved development, growth, body taurine and survival rates and increased amylase and trypsin activities during early larval stages. Taurine supplementation also resulted in higher enzyme activities for amylase, lipase, trypsin and pepsin. In addition, when larval red sea bream P. major (Chen et al. 2004), Japanese flounder P. olivaceus (Chen et al. 2005), Pacific cod Gadus macrocephalus (Matsunari et al. 2005b), California yellowtail and white sea bass Atractoscion nobilis (Rotman et al. 2012) were fed taurine-enriched rotifers, larval growth, survival and body taurine were significantly improved compared with taurinefree rotifers.
Supplementing microencapsulated diets with taurine may also improve marine larval performance and survival. Takeuchi et al. (2001b) found that larval red sea bream (P. major) fed with a microparticle diet supplemented with taurine, together with rotifers, their body taurine was significantly increased. Pinto et al. (2010) also evaluated the effect of dietary taurine-supplemented microcapsules (during the pelagic phase) and artemia (during newly settled phase) on Senegalese sole (Solea senegalensis) larvae. Taurine supplementation did not affect larval growth and metamorphosis during the pelagic phase. However, sole larvae previously fed microcapsules supplemented with taurine had higher growth rate and metamorphosis success than larvae fed control microcapsules. This particular finding supports the assumption that previous feeding history affects taurine synthesis and requirement in fish.
On the contrary to the above results, which clearly indicated the necessity of dietary taurine for larval development of several marine fish species, dietary taurine supplementation does not seem to enhance the larval growth performance and survival of gilthead sea bream (Sparus aurata) (Pinto et al. 2013). When the larvae were fed rotifers supplemented with taurine-enriched liposomes, growth performance, survival or larval taurine levels were not significantly affected. When the larvae fed with live prey were subsequently fed a solution containing L-methionine with or without supplemental taurine, dietary taurine supplementation led to an increase in body methionine retention. This finding suggests that gilthead sea bream larvae have the ability to synthesize taurine (through the conversion of methionine into taurine). However, it would be unwise to build a conclusion on whether or not taurine is essential for larval gilthead sea bream based on only one study. More work is urgently needed to support this finding.
Conclusion
The present review showed that taurine synthesis widely differs between fish species, depending on fish species and size, feeding habits and cysteinesulphinate decarboxylase (CSD) activity. Generally, freshwater species have better ability of taurine synthesis than marine species. However, some freshwater species have limited ability of taurine synthesis, and they require exogenous taurine source, especially if they are fed protein sources of plant origins. Marine fish species have low or negligible ability to synthesize taurine due to a limited, or lack of, activity of CSD during intermediate metabolism from methionine to cystathionine. Therefore, taurine is conditionally essential when these fish are fed diets containing high plant protein levels and deficient in methionine and/or cysteine, especially during early life stages. Under such conditions, taurine supplementation would be necessary for optimum growth rates, feed efficiency, body taurine and physiological functions. Live food or microcapsules enriched with taurine can also improve larval morphology, development, growth and survival of marine fish and shrimp. Taurine also plays significant osmoregulatory, simulative, protective and antioxidative roles in fish and shrimp, in addition to its important role in retinal development and visual system.
September 2013