Coastal marine invertebrates are exposed to dissolved carbon dioxide levels that fluctuate on time scales ranging from daily to seasonal as a result of both natural processes and human activities. These aqueous CO2 levels are likely to increase (and pH to drop) in the decades ahead as a consequence of ocean acidification (OA), the uptake of anthropogenic CO2 by the ocean. Since pre-industrial times, increases in anthropogenic emissions of CO2 have caused a decrease in surface ocean water pH of 0.1 units, and a decrease of another 0.2–0.3 units is projected by the end of this century. As seawater pH decreases, its calcium carbonate saturation state (Ω) also decreases, and drops in Ω have the potential to make calcification (or shell-building) more difficult, or more energetically costly for the organism.
While changes in surface ocean pH are happening on a global scale, the processes affecting coastal and estuarine ocean pH are different and result in more seasonably variable conditions than in the open ocean. Anthropogenic eutrophication is a major factor influencing acidification of coastal and estuarine regions. Eutrophication results in algal blooms, which eventually die, sink to the seafloor, and fuel microbial respiration. This process is exacerbated during summer stratification events and can produce low-pH seasonal bottom waters that are undersaturated with respect to aragonite. Because many coastal bivalve species spawn during summer months, the larvae are exposed to such conditions. Furthermore, because the buffering capacity of seawater is reduced as dissolved inorganic carbon (DIC) increases, it has been suggested that eutrophication could increase the susceptibility of coastal waters to ocean acidification. As atmospheric CO2-driven OA and anthropogenic eutrophication increase, the conditions experienced by bivalve larvae will become increasingly unfavorable for shell growth.
There is mounting evidence for negative effects of OA on marine organisms that produce calcareous skeletons, shells, or tests. In particular, marine invertebrate larvae, including bivalves, are vulnerable to a variety of both chemical and physical environmental conditions including decreased pH. For example, mussel and oyster larvae raised in water with pH ~ 7.4 were shown to have delayed development to D-stage veligers compared to larvae raised in ambient pH ~8.1. Talmage and Gobler showed that hard clams (Mercenaria mercenaria) and, to a lesser extent, bay scallops (Argopecten irradians) experienced delayed metamorphosis at present-day conditions compared to pre-industrial conditions, suggesting that the 0.1 pH decrease in the last 150 years has already had an impact on bivalve species. OA has been shown to have a negative impact on multiple early life stages of bivalves, including fertilization, D-stage (early) development, later larval development, and juvenile development. Some species appear to be more tolerant of OA conditions than others. For example, two species of oysters displayed different trends when exposed to a range of pCO2 values resulting in Ωar= 1.3–0.6 for ~30 days; Crassostrea virginica had decreased shell area and decreased calcification with decreasing Ωar, but Crassostrea ariakensis showed no change in shell area or calcification. Nonetheless, the majority of work has demonstrated that young bivalves are negatively impacted by the high CO2/low pH conditions resulting from ocean acidification. There is evidence that the early larvae of some species are susceptible to OA. Additionally, OA conditions have been shown to negatively affect the survival of bay scallop larvae and the size of competent and post-metamorphic bay scallops.
Here, we address the impact of early exposure to elevated CO2 on the survival and growth of bay scallop larvae. Bay scallops are ideal as a model organism for this study because of their economic importance as a commercially harvested shellfish and because of their relatively short larval duration (~2–3 weeks). We also investigate whether transferring the larvae to ambient conditions can reverse the effects of early high CO2 exposure. Such a scenario is ecologically relevant in a situation where larvae are spawned in an estuary with relatively high pCO2 and are subsequently transported by currents or tides out of the estuary to sites with a lower pCO2. We exposed larvae to ambient (nominally 390 ppm CO2, pH= 7.93) and high (nominally 2200 ppm CO2, pH= 7.39) CO2 conditions for a total of 18 days. The high CO2 treatment produced a calcium carbonate saturation state that was undersaturated with respect to aragonite (Ωaragonite). Such pCO2 values and associated saturation states have been observed in summer months in a local estuary (Childs River, Falmouth, MA, USA) where bivalve larvae are found. In addition, we exposed a third group of larvae to high CO2 conditions for three days (through the larval D-stage), followed by exposure to ambient CO2 conditions for 15 days. We hypothesize that larvae exposed continuously to elevated CO2 will grow and develop more slowly throughout the larval period than those exposed to ambient CO2 and that survival will also be negatively affected throughout the larval period. We explore whether effects of exposure to elevated CO2 conditions during critical initial shell-formation (1–3 days post fertilization) can be altered by a return to ambient CO2 conditions.
Results
Shell Length
Larval development (Fig. 1) in all treatments progressed in a sequence typical for this species as described by Belding and Widman et al. All larvae were fully shelled at 1 d and were post-D-stage by 3 d. While fully shelled, some 1 d larvae had velums that protruded from the shell (Fig. 1d, g); this occurred more frequently in larvae from the High CO2/ Ambient and High CO2 treatments than in larvae from the Ambient CO2 treatment.
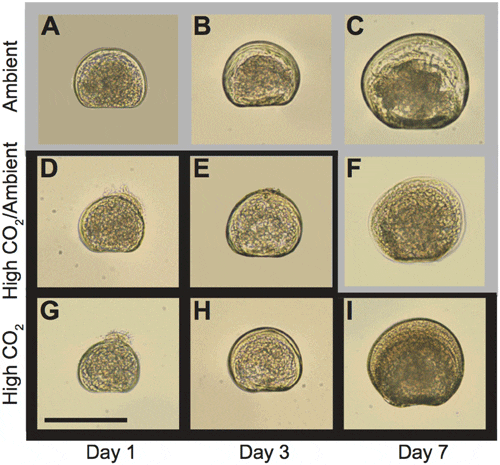
doi:10.1371/journal.pone.0061065.g001
Exposure to high CO2 (Table 1) caused a significant reduction in shell length (Fig. 2) during the first week of development when compared to exposure to ambient CO2 (repeated measures ANOVA, Wilk’s Lambda= 0.033; F=19.47; df = 6, 26; p<0.00001). This pattern was significant for each of days 1, 3, and 7 (One-way ANOVA; Table 2). On day 1, after 12 h of exposure, the mean shell lengths of all three treatments were significantly different from each other (Fig. 2). The difference on day 1 between the High CO2/Ambient and High CO2 treatments was unexpected, as the larvae were in similar conditions prior to the switch on day 3. On days 3 and 7, the mean shell lengths of larvae from the High CO2 and High CO2/Ambient treatments were no longer significantly different from each other, despite having been in different conditions since day 3. The mean lengths of shells from the High CO2 treatment were 84.1 per cent, 92.5 per cent, and 88.5 per cent of the mean lengths of shells from the Ambient CO2 treatment on days 1, 3, and 7, respectively. After day 1, mean (±1 standard deviation) shell growth rates integrated over following 6 days were 7.4±1.5 μm d21 for the Ambient CO2 treatment, 6.7±1.2 μm d21 for High CO2/Ambient, and 7.2±1.0 μm d-1 for High CO2; these differences were not significant (one-way ANOVA, F =0.50, df =2, p= 0.62).
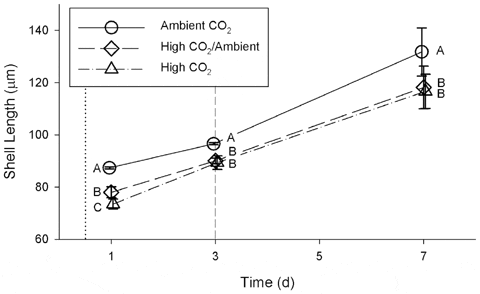
doi:10.1371/journal.pone.0061065.g002
Larval Survival
Survival of scallop larvae in the Ambient CO2 treatment was consistently higher than survival of scallop larvae in either the High/Ambient or High CO2 treatments (Fig. 3) during the first week of development. This overall effect on larval survival was significant in a repeated measures ANOVA (Wilk’s Lambda = 0.38; F =2.50; df= 6, 24; p=0.05). However, when the effect was examined for individual days, survival in the Ambient CO2 treatment was significantly higher only on day 1 (Table 3, Fig. 3). Survival of larvae in the High/Ambient CO2 treatment was not significantly different than in the other treatments at any time during the first week of development.
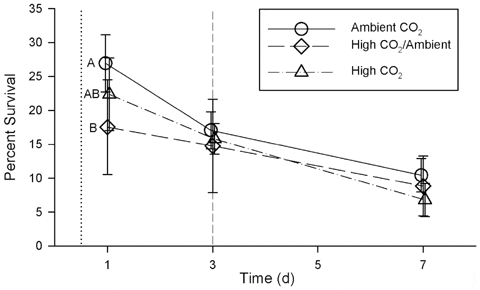
doi:10.1371/journal.pone.0061065.g003
Discussion
Early exposure (12–24 hours post-fertilization) to high CO2 significantly reduced larval shell size (Fig. 2) and survival (Fig. 3) relative to ambient CO2 by the time the larvae were 1 d old. The initial reduction in size relative to the Ambient CO2 treatment was still evident after the first week of larval development. This suggests that CO2 exposure during the first day is critical to shell development. Growth rate from 1–7 d was not significantly affected by CO2 exposure, further indicating that growth during the first 24 h post-fertilization determines shell size later in development – the larvae did not increase their growth rate to compensate for initial slow growth. There is evidence that some bivalve larvae (Pacific oysters, Crassostrea gigas, and hard clams, M. mercenaria) use amorphous calcium carbonate (ACC) to produce the earliest stages of their shell. If this is also true for bay scallops, then it may be a factor in explaining the sensitivity of bay scallop larvae to high CO2 conditions during the first day of development, as ACC is more soluble than aragonite and its formation would therefore be less thermodynamically favorable. This demonstration of a significant and lasting CO2 effect on shell size within the first day of larval development suggests that other studies on bivalve larval development in which CO2 exposure was initiated after the first day of larval development and initial calcification may have underestimated the magnitude of the effects of high CO2 throughout larval development.
Exposure to high CO2 caused a significant decrease, relative to ambient conditions, in larval survival at 1 d post fertilization, and a consistent, but not significant, decrease at 3 and 7 d post fertilization. Survival of <20 per cent of individuals on day 7 is low compared to other studies of this species, probably due to a combination of not using antibiotics, and calculating survival from initial counts at 12 h post-fertilization rather than 3 d. Mortality during the first 3 d of larval development typically is high and can be variable between culture vessels even when conditions are held constant (Widman, J.C. Jr., personal communication). In our study, survival was highly variable among replicates within treatments and was likely influenced by factors other than carbonate chemistry. This variability may contribute to unexplainable patterns in survival such as the differences on day 1 between High CO2 and High CO2/Ambient treatments, despite the similar conditions.
A size reduction in scallop larvae exposed to high CO2 may have indirect effects on subsequent survival in the field. The age at which a scallop larva is competent to metamorphose is often affected by size. Small scallop larvae may delay metamorphosis, increasing their time in the plankton and risk of mortality from planktonic predators. Previous work has found that exposure of bivalve larvae to high CO2 treatments cause delayed metamorphosis, although it is not clear if small size was the cause of the delay. In addition, if the smaller size of bay scallop larvae exposed to high CO2 persisted through metamorphosis into adulthood, the reproductive output of those individuals could be stunted simply because the size of the gonad is proportional to the size of the scallop.
The negative effect of high CO2 exposure on bay scallop larval size is consistent with negative effects of high CO2 exposure on the size of other larval bivalves. The clam Macoma balthica has been shown to produce significantly smaller larval shells at 3 d when exposed to seawater with a pH of 7.5 or 7.8 directly after fertilization, compared to a control treatment with pH 8.1. The same group also reported a similar trend in which M. balthica larvae produced (non-significantly) smaller shells from day 5 to 19 when exposed to lowered pH. Similarly, larvae of the blue mussel, Mytilus edulis, had shells that, at 2 d, were 12.7 per cent smaller when raised in water with pH 7.6 compared to larvae grown in water with pH 8.1. Larvae of the oyster Saccostrea glomerata showed at 8 d a decrease in shell size of 6.3 per cent at pH 7.8 and 8.7 per cent at pH 7.6 relative to pH 8.1. By documenting a size effect in the early larval stage of bay scallops, we can better understand the Talmage and Gobler observations of negative effects of high CO2 exposure on shell size of 19–20 d old competent scallops. Our results suggest that a decrease in size of competent larvae and set juveniles exposed to high CO2 may be a lingering effect of compromised growth in early stages.
Recent work has suggested that marine organisms vary in their potential for evolutionary change in response to high CO2 conditions. For example, when exposed to high CO2 conditions (2200 ppm), coccolithophore (Emiliania huxleyi) clones that had been raised in high CO2 conditions for 500 generations showed a reduced negative calcification response when compared to clones that had been raised in ambient CO2 conditions for 500 generations. This indicates that the clones exposed to high CO2 conditions for many generations adapted to the unfavorable conditions. Similarly, Sunday et al. showed that larvae of the sea urchin Strongylocentrotus franciscanus may have faster evolutionary responses to high CO2 than larvae of the mussel Mytilus trossulus, despite the fact that M. trossulus has a faster generation time and breeder replacement time. Studies of the evolutionary potential of marine invertebrates to adapt to high CO2 conditions have been initiated only recently, and bay scallops may be a useful model organism for trans-generational laboratory culturing experiments, as they reach sexual maturity in just one year.
Acidification of coastal waters is affected by atmospheric CO2 levels, but it is also strongly impacted by eutrophication, mixing and circulation, and input of fresh water. We showed a CO2 exposure effect on larval bay scallop growth, but we did not include hypoxia as a treatment. Hypoxia typically co-occurs with high CO2 (hypercapnia) as a result of eutrophication in coastal and estuarine waters. Because hypoxia and hypercapnia act synergistically on the responses of marine invertebrates found in such environments, it is possible that the interaction of low oxygen and high CO2 will affect larval bay scallop development even more strongly than high CO2 alone.
The larvae in both High CO2 and Ambient CO2 treatments maintained similar growth rates from 1–7 d, but the negative effects on shell length of exposure to high CO2 during the first 3 d of development were still present after a week of larval development. This result suggests that there is a critical initial window in which CO2 exposure is particularly damaging to scallop larvae. Larvae that spawn in coastal and estuarine environments when pCO2 is high may have smaller shells, even if they are transported within a few days to waters with lower CO2. Aquaculture facilities may need to monitor pCO2 in the water used for rearing early larvae to ensure that conditions do not impair growth.
March 2014