From the extensive culture of James River oyster seed in lower Chesapeake Bay beginning in the 1800s, to the intensive culture of millions of oysters and clams throughout the region today, the production of molluscan shellfish has a long and important history in the southeastern United States. Now, however, disease is a major threat to cultured shellfish, especially to oysters.
The causes of some disease losses—such as winter mortality in clams and summer mortality in oysters—are elusive. But some diseases are better understood. These include dermo, MSX and SSO diseases in the oyster Crassostrea virginica, and QPX disease in the hard clam Mercenaria mercenaria. This publication addresses the management of these infectious diseases.
Dermo Disease in Eastern Oysters
Dermo disease in C. virginica is the most significant molluscan disease in the southeastern region. It is caused by the native parasite Perkinsus marinus (originally described as Dermocystidium marinum, to which the abbreviation “dermo” refers). Protistan parasites of this genus infect molluscs in warm waters around the world. Perkinsus marinus occurs throughout the Gulf of Mexico and along the Atlantic coast north to New England. It causes serious mortality in all but the northernmost part of its range.
Perkinsus marinus is directly transmitted between oysters, primarily when oysters with advanced infections die and disintegrate. New infections are acquired as oysters feed, the parasite infecting its hosts through gut epithelia. Infections may remain in the gut epithelium but often become systemic, resulting in significant destruction of epithelia and connective tissues (Fig. 1).
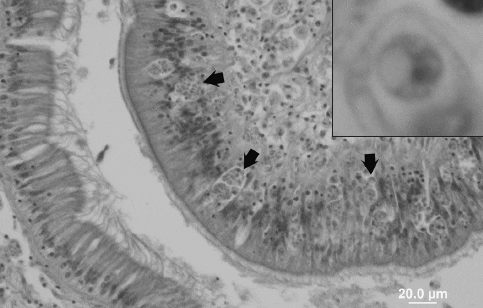
This destruction is partly caused by the digestion of host tissues by enzymes the parasite secretes. Affected oysters are typically thin, pale and “wasted” in appearance, as tissues are overrun by explosively proliferating parasites. Uninucleate trophozoites of 2 to 10 ?m develop into multinucleate “rosette” forms (to 15 ?m) that rupture to release more uninucleate trophozoites. This process occurs most rapidly and causes the greatest mortality during the warm summer and early fall months. P. marinus proliferates at temperatures higher than 20 °C, but most rapidly at 25 °C and higher. In warm, southern waters dermo may kill more than 50 percent of oysters each year.
P. marinus thrives at estuarine salinities. Warmseason salinities as low as 12 ppt will support full dermo disease outbreaks, so areas most at risk can be identified by local salinity patterns. Salinity is a key determinant of the local distribution of dermo disease. P. marinus does tolerate periods of low salinity, however. Even at salinities below 5 ppt the parasite can survive for extended periods. So pulses of low salinity produced by typical summer storms are unlikely to purge P. marinus from oyster populations, but fluctuations in salinity do influence the timing of intense dermo disease outbreaks. The most serious outbreaks are associated with drought years. Seasonal morbidity and mortality are typically delayed to later summer or fall in systems greatly influenced by rivers, which generally flow most strongly and produce the lowest estuarine salinities in the spring. In the Gulf of Mexico, dermo outbreaks may be linked to the El Niño – Southern Oscillation (ENSO) cycle, with serious outbreaks regularly following the arrival of warm, dry, La Niña climatic conditions.
The effect of dermo disease in aquaculture can be minimized by culturing oysters in waters where the autumn salinity is below 12 to 15 ppt. Prospects for successful culture in waters of higher salinity are improving with the development of fast-growing, relatively disease-resistant, domesticated oyster strains. While oysters of these strains acquire P. marinus infections, many reach market size before suffering serious morbidity and mortality, even in waters of higher salinity (more than 15 ppt). Because the risk of dermo disease increases with the age of oysters, decreasing the time to market is fundamental to profitable oyster aquaculture. The fallowing of P. marinus-infected commercial oysters beds was recommended years ago in Virginia, but it is not clear that this strategy would be beneficial anywhere today, given the abundance of this parasite. And because transmission can occur over long distances (kilometers), siting oyster production far from sources of infection such as reefs or structures harboring wild oysters will not necessarily protect oyster crops from dermo disease.
MSX Disease in Eastern Oysters
MSX disease in C. virginica is caused by the protistan parasite Haplosporidium nelsoni. MSX refers to Multinucleate Sphere Unknown, as this pathogen was known before its description in 1966. Haplosporidium nelsoni was introduced from Asia and is notorious as the cause of catastrophic (more than 90 percent) oyster mortality in Delaware Bay beginning in 1957 and in Chesapeake Bay beginning in 1959. These were the first catastrophic oyster disease outbreaks in the region. In Chesapeake Bay, because of continued overharvesting, the spread of dermo disease to lower salinity waters in the 1980s, and the general deterioration of the Bay environment, oyster populations have never completely recovered.
Haplosporidium nelsoni has been documented along the Atlantic coast from Nova Scotia to Florida, with epizootic disease and significant mortality occurring from Maine to Virginia. It is apparently not a serious cause of mortality south of Virginia, for reasons that are not known. A recent molecular survey hinted at the presence of H. nelsoni in the Gulf of Mexico, but its presence there has never been visually confirmed. Seed produced from Gulf oysters are highly susceptible to MSX disease when cultured in Mid-Atlantic waters, which suggests that Gulf populations have never been exposed to MSX.
Unlike P. marinus, H. nelsoni is not directly transmissible among oysters. The intermediate host or hosts that are thus presumed to be involved in H. nelsoni transmission have never been identified. Susceptible oysters become infected from some unknown environmental source and die from May through October in the Chesapeake region. Initial infections occur in the epithelia of the gills and palps. From these epithelia, H. nelsoni colonizes connective tissues and large, multinucleate H. nelsoni plasmodia (to 50 ?m; Fig. 2) spread throughout the host.
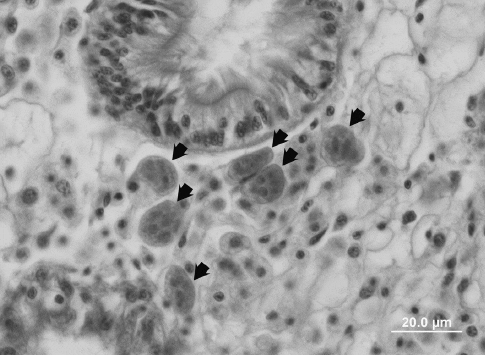
Spores, presumably a cell form essential to transmission of the parasite, form in the digestive tubules, primarily of younger oysters.
Salinity plays a key role in determining the distribution of H. nelsoni. The parasite cannot withstand salinities below 10 ppt for more than 2 weeks when temperatures are above 20 °C, so periods of low salinity, particularly in the warmer months, effectively purge MSX from oyster beds. Following Tropical Storm Agnes in June 1972, for example, H. nelsoni disappeared from most Chesapeake Bay oyster populations. The effects of temperature are more subtle and complicated. Studies in Delaware Bay indicated that although H. nelsoni can proliferate rapidly at temperatures from 5 to 20 °C, resistant oysters can manage H. nelsoni at higher temperatures while susceptible oysters cannot. The apparent insignificance of H. nelsoni south of Virginia—and its absence from the Gulf—may relate partly to temperature, in that warm southern water may favor the oyster’s defenses over the parasite. The distribution of MSX disease may also be influenced by the distribution and abundance of H. nelsoni’s unknown intermediate host(s).
The management of MSX in disease-enzootic waters begins with the use of disease-resistant oyster stocks, which have been in development since the 1960s. These oysters either resist infection entirely or are able to restrict parasites to gill and palp epithelia. Few resistant oysters are killed by MSX. Even wild oysters from the Mid-Atlantic have developed some MSX resistance; very little MSX disease is observed in adult oysters in the Mid-Atlantic, while naïve oysters transplanted from low salinity waters are greatly affected. Aquaculturists in the Gulf of Mexico should avoid introducing H. nelsoni from Atlantic waters.
SSO Disease in Eastern Oysters
SSO disease in C. virginica is caused by the protistan parasite Haplosporidium costale, a relative of the MSX disease agent H. nelsoni that generally occurs in higher salinity (? 20 ppt) coastal waters. SSO refers to Sea Side Organism, as this protist was first known. It was discovered in Virginia’s coastal sounds during the initial investigations on MSX, and is now known to range north from Virginia to the Atlantic provinces of Canada.
In Virginia waters, H. costale parasitism and the associated SSO disease and mortality display a distinct seasonality, peaking sharply in April-June of each year. New infections at this time coincide with the deaths of previously infected oysters, though transmission is still thought to occur through some intermediate host(s). Infection probably occurs through digestive tubules, with multinucleate parasite plasmodia (like those of H. nelsoni, but smaller, to 10 ?m) then proliferating in connective tissues. Spores form in the connective tissues (Fig. 3).
While SSO disease has been reported to cause more than 50 percent mortality, it is unlikely that it does so today, at least in Virginia waters. While a maximum of 56 percent of adult oysters were infected by H. costale at one Virginia site in April 2008, the percentage of advanced infections was just 4 percent and the parasite could barely be detected (4 percent prevalence) 1 month later. Compared with P. marinus, H. costale is a minor pathogen in the region today. Where it does occur, planting fastgrowing oyster seed after the peak infection period (April- June) should ensure minimal problems the subsequent spring, and allow oysters to grow to market size before serious problems develop the following year.
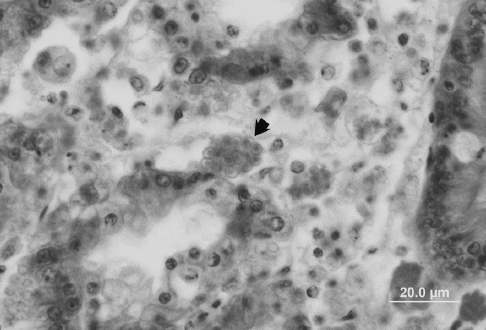
QPX Disease in Hard Clams
Infection of the hard clam M. mercenaria by the protist QPX (Quahog Parasite Unknown, Fig. 4) has been known since this parasite emerged in 1989 in Prince Edward Island, Canada, and in 1991 in Massachusetts.
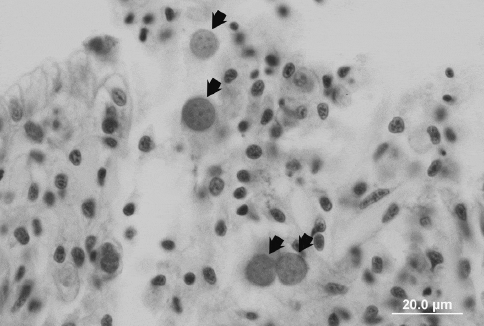
The parasite was probably present along the Atlantic coast much earlier. Its southward distribution extends to Virginia, where it has caused occasional mortality.
QPX infections are usually localized at the mantle and gills, where various cell forms 2 to 48 ?m in diameter, typically surrounded by clear halos, can be observed disrupting connective tissues. Infections can become systemic and sometimes result in serious mortality. Eighty percent of the clams in some affected beds have been lost. It is not certain how QPX infections arise. Labyrinthulid protists, the group to which QPX belongs, are ubiquitous saprophytes, meaning that they feed on dead or dying material. It is possible that QPX is a typical saprophytic labyrinthulid that, under certain conditions, also functions as a pathogen. That is, it may be capable of living externally in the environment or internally as a pathogen in clams. While we do not completely understand the cause of QPX outbreaks, clam culturists in Virginia avoid exceptionally high densities, a potential source of stress on the clams, and prevent excessive fouling of nets because the fouling algae are a potential environmental source of the parasite.
It is possible, but not certain, that warm water temperatures limit the southward distribution of QPX. It does appear that estuarine salinities are inhospitable to the parasite, as QPX has rarely been observed in Chesapeake Bay. As QPX is not broadly distributed within the region, local clam stocks south of Virginia or in waters below 20 ppt may never have been exposed to QPX. Indeed, clam stocks originating south of Virginia are very susceptible to QPX when grown in northern waters. For this reason, clams from QPX-enzootic waters, which presumably are resistant to QPX disease, should be used in areas where QPX occurs, even if seed is to be produced in more southern clam hatcheries.
Introduction of Exotic Diseases
The arrival of exotic molluscan pathogens is impossible to predict. The likelihood of a pathogen introduction may have decreased somewhat in recent decades because the deliberate transplantation of exotic molluscan hosts has slowed and surveillance for disease has improved. Yet the risk of an accidental introduction via shipping traffic (hull fouling, ballast water, etc.) persists, and other vectors have become apparent, such as the import of exotic molluscs for the ornamental trade. The parasite Perkinsus olseni was recently detected in Florida in giant clams imported from Vietnam for ornamental use. This raises concerns that the release of unwanted aquarium species by hobbyists could bring dangerous pathogens to the region. Careful disease management by the ornamental fish and shellfish industry is imperative.
With the rejection of efforts to introduce the Suminoe oyster Crassostrea ariakensis to Virginia waters, it is unlikely that an exotic mollusc will again be cultured in open waters of the Southeast anytime soon. Nonetheless, hatchery work with non-native species may continue or expand for the international sale of larvae and seed. Imported, exotic broodstocks should be carefully quarantined to prevent the introduction of exotic pathogens, including herpesviruses.
Aquaculture Health Management
While some aspects of aquaculture health management are specific to certain disease problems, others are universal. At the farm level, health management begins with creating conditions that minimize stress on cultured animals and with attention to site selection, farm organization, planting densities, and routine husbandry to ensure good nutrition and growth. It is important to use genetic strains of oysters and clams that exhibit good growth and survival under local conditions. Cultured molluscs should be monitored for unusual reductions in growth, condition or survival. If any of these situations occurs, pathological examinations should be performed to identify problems such as emerging disease at the earliest stage.
At a broader level, the diversity and distribution of pathogens should be determined so that disease-enzootic regions can be better defined. Stocks destined for transplantation should be screened to ensure that diseases are not spread to new areas. The International Council for the Exploration of the Sea (ICES) Code of Practice on the Introductions and Transfers of Marine Organisms describes procedures for safe transplantation. Where there is a risk of introducing a disease, culturists are advised to plant only certified disease-free progeny produced in quarantine from introduced broodstock.
New fast-growing, disease-resistant oyster and clam stocks should be developed for regional use, and the natural diversity of aquaculture species should be conserved. It is the diverse wild populations that are capable of evolutionary response to new disease and environmental challenges, and from which new aquaculture lines may continually be developed. Finally, the diversification of the aquaculture industry to include new local species should continue. An industry with a broader base of cultured species should be better able to withstand the effect of disease on any one.