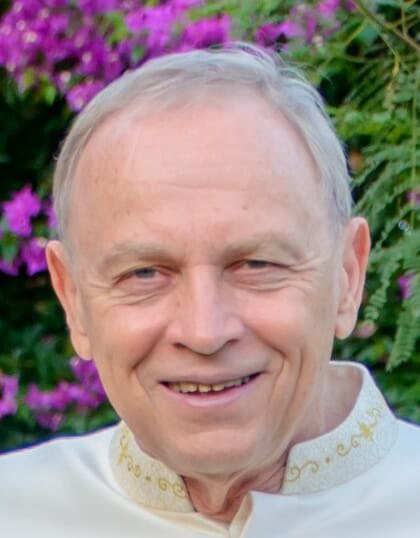
In the late 1970s, Dr James Rakocy was finishing his PhD at Auburn University, Alabama. His research focused on growing plants with fish, creating an ecosystem where nutrients from the fish provided fertiliser for the plants, while the plants absorbed all the waste nitrogen and phosphorous excreted by the fish, which kept the water crystal clear and free of algae.
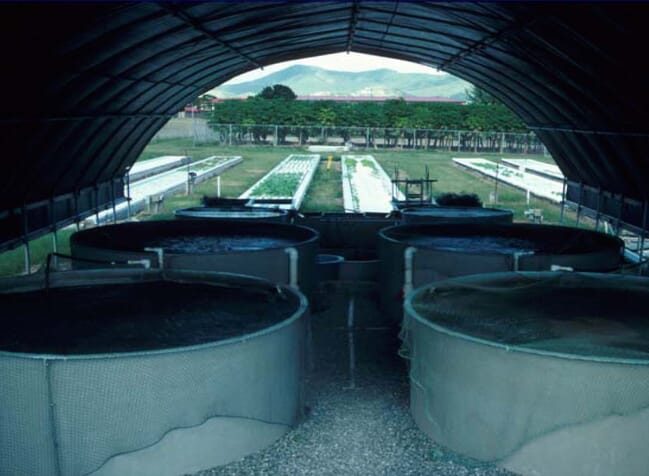
Dr Rakocy's first commercial sized system, which was composed of four fish-rearing tanks and six growing beds. © Dr J Rakocy
During the 1980s, Dr Rakocy went on to develop a commercial aquaponics system at the University of the Virgin Islands (UVI) and, over the course of the next 30 years, he and his team perfected a commercially viable aquaponics system that allows regular harvests of fish and plants without water exchange and a very moderate amount of supplemental nutrient input. The final system design only required the regular addition of calcium and potassium hydroxide to keep pH levels at 7.0, and every three weeks the plants needed the addition of iron.
The evolution of the aquaponic system
According to Dr Rakocy, his initial setup at UVI, using 55-gallon drums, had shown that a system integrating plants and fish could work successfully over a long period of time, with little nutrient input to sustain plant growth.
The system’s components were a gravel bed, where plants were growing, a conical filter settling tank for the collection of larger, settleable solid waste and a separate tank where the fish were kept. In this case, and in future experiments, tilapia proved the ideal candidate fish.
The only problem with this original design was that the gravel used as a medium for the plant roots got clogged regularly with suspended solid waste from the system. The conical clarifier proved to be very effective at removing settleable solids and would remain an important component of this system. To control suspended solids and dissolved organic nutrients, a simple but ingenious filter, composed of bird netting in a long rectangular tank, would prove viable from both a biological and economic standpoint.
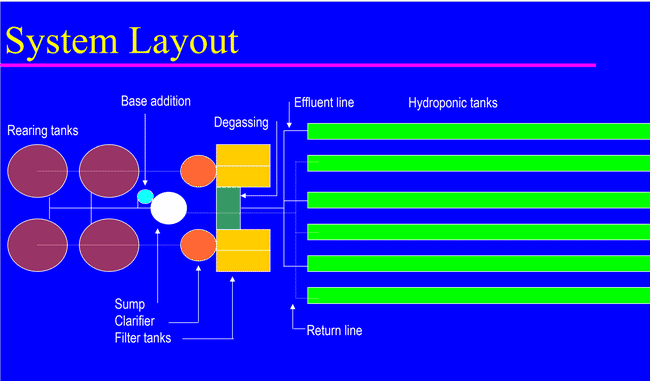
© Dr J Rakocy
The gravel growing beds were replaced by floating rafts, a system known as deep-water culture (DWC). To avoid clogging, the addition of thin plastic bird netting collected suspended solids. Without it, solids would settle in the growing beds, resulting in denitrification, a process that removes the nitrate that the plants need for growth. Moreover, denitrification would produce alkalinity, eliminating the opportunity to add calcium and potassium hydroxide to increase the pH. Calcium and potassium are the two elements regularly added to prevent plant mineral deficiencies, so solid accumulation in the tanks that housed the rafts had to be eliminated in order to deliver calcium and potassium to plants in the hydroxide form.
In addition, without this novel suspended-solids filter, dissolved organic nutrients would build up and result in bacterial slime covering the plant roots, reducing plant growth and perhaps even killing the plants. The organic matter that accumulated on the bird netting underwent bacterial decomposition, a process known as mineralisation. This released nutrients from the organic matter in an inorganic form that is required for plant growth, as their roots can only absorb nutrients in an inorganic form. The major nutrients released from the organic matter are nitrogen and orthophosphate. This form of phosphorous is useable by plants and its release is a very important job performed by this novel filter. The netting biofilter needs to be periodically removed and cleaned with a high-pressure hose to remove the accumulation of organic matter; this proved to be a water-quality management tool which could be used to control the nitrate levels in the system. Frequent cleaning of the filter decreases solids accumulation and denitrification, resulting in higher nitrate levels in the system for the production of leafy green vegetables, like lettuce. Less frequent cleaning increases solids accumulation and denitrification, resulting in lower nitrate levels in the system for increased production of fruiting plants, like tomatoes.
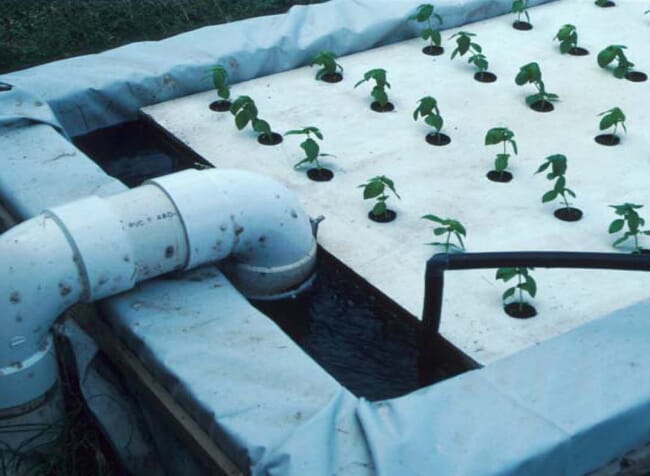
© Dr J Rakocy
After the system was scaled up (one 3,000-gallon fish tank and two 74-ft2 growing beds) and replicated six times, the fish-stocking-rate to plant-growing-area ratio proved to be incorrect, with too much nitrogen and other nutrients accumulating, and not enough plants available to absorb the nutrients. An experiment was conducted in the six systems to determine the optimum feeding-rate ratio – ie the amount of fish feed per plant growing area per day – which was 60 grams of feed input per square metre of plant growth area. This ratio was ideal to prevent nutrient accumulation or nutrient deficiencies and was used throughout the development of future systems at UVI.
This experimental system was then scaled up to a commercial size, in a site composed of four fish-rearing tanks (2,060 gallons each) and six growing beds (384 square feet each). Production was staggered, with one tank harvested and restocked every six weeks. The growing period for each tank was 24 weeks. Production of lettuce and other leafy green vegetables was staggered too, so that 25 percent of the plants were harvested every week. The growing period for lettuce seedlings was four weeks. By staggering fish and plant production, nutrient input from the fish feed and nutrient uptake by the plants were relatively constant. This system allowed the continual harvest, and sale, of fish and plants.
The quest for commercial viability
Once these problems were solved, the next step was to establish a market so this system could become commercially viable. When questioned about this Dr Rakocy related the necessity of two important factors:
1) The aquaponics system should be large, in the order of about 1 hectare, in order to benefit from an economy of scale.
2) The aquaponics system should be located near a large market, so that harvestable products could be sold directly to consumers, increasing the profit potential by eliminating the need for wholesale distribution.
Dr Rakocy stated that current commercially viable aquaponic operations, such as Superior Fresh, are successful because they are large and indeed located close to a large market, using lettuce as the plant species and adding value by selling it as a cut and bagged product. Moreover, leafy greens should be used as they are the most profitable component in an aquaponics system, with a high turnover rate, being harvested every month, as well as enjoying a wide acceptance in the marketplace. Additional profitable plants used in aquaponics systems include herbs, such as basil, which grow fast and can be partially harvested to continually supply markets.
For commercial production, says Dr Rakocy, multiple systems increase biosecurity, as any disease problems can be contained. By producing all of the needed tilapia fingerlings and plant seedlings on site, disease introduction from hatcheries and nurseries can be excluded, making aquaponics systems more sustainable.
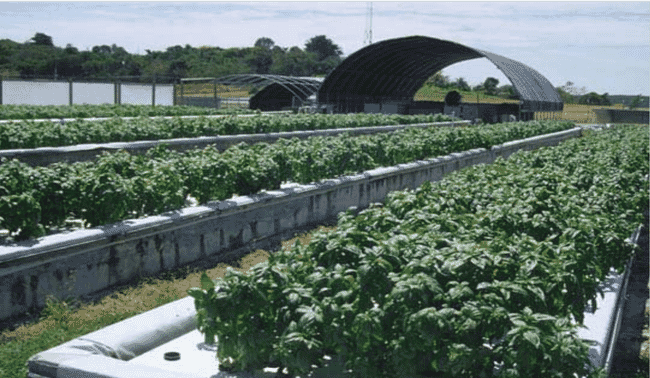
© Dr J Rakocy
Another advantage of the UVI aquaponics system is that water exchange is less than 1 percent of the water input per day. This means that the system maintains a low, regular level of dissolved and particulate organic matter, which provides the additional benefit of producing very healthy, faster-growing, disease-resistant plants. Aquaponic plants are more resistant to pests than plants in hydroponic systems, which do not have the benefit of organic compounds and rely solely on inorganic nutrients. Hydroponic systems produce plants that are often more stressed, which creates a higher sugar content in the leaves, which in turn attracts pests. Hydroponic systems also have the disadvantage of developing unbalanced nutrient concentrations, which require continual adjustment. Eventually hydroponic solutions need to discarded and replaced with new nutrient solutions. In contrast, once aquaponic systems are established, they become stable enough that regular measurement of nutrients is not even necessary.
Looking to the future
Dr Rakocy notes that research in aquaponics is currently being undertaken in many countries. New approaches are being studied, as well as the use of different high-value fish and plant species. In addition to the expansion of commercial operations, aquaponics is very popular now with hobby farmers, who are producing a large variety of plants and fish in multiple design systems that use locally available materials and new construction methods. According to Dr Rakocy, given the wide range of interest in aquaponics, its future looks bright.