Introduction
To date, the trout industry in general has not adopted photoperiod techniques for production enhancement. Extensive research over the last seven years at Stirling has shown the potential benefits of such regimes. This article summarises previous and ongoing research determining how artificial photoperiod regimes can be used to manipulate reproduction and growth for the benefit of the trout industry. The most important advances for farming relate to our improved understanding of light perception which, in conjunction with technological innovations, has allowed radical refinement of the available lighting systems.
Light Perception
The two main areas concerning the key physical properties of light are intensity and spectral content.
Light Intensity
It is now believed that light intensity per se is not the key factor in photoperiod manipulation of fish, but rather the generation of diffuse lighting fields throughout the culture system that allow the fish to perceive the artificial light whatever their position. In both tank and cage systems for ongrowing fry and portion-size fish, constant illumination using two light units (400W submersible metal halogen) within the system increased growth above that achieved using one light unit, although both light treatments grew faster than fish under ambient light (Figure 1A & B).
Light intensity measurements revealed that in the two light treatment there was a slightly higher and, more importantly, a more uniform light distribution. This led to a more consistent response from the population and less variation in size between individuals. Improving growth rate has numerous benefits including:
- greater production turnover
- capacity for different fry stock-out times allowing optimal use of facilities and operation
- attainment of harvest size sooner prevents market saturation and premium prices to be maintained
- a more efficient FCR results in reduced economic waste and environmental impact.
Currently within aquaculture, the tendency is towards the use of more numerous and more powerful underwater lighting units. However, such high intensity point sources make light management awkward and energy consumption expensive, and are therefore not viable options for profitable farming. Lighting systems suitable for trout farming therefore require radical remodelling with the biological sensitivities of the species being the focal point for system design, coupled with cost-benefit analysis. Studies performed in Stirling are determining these species-specific light intensity thresholds.
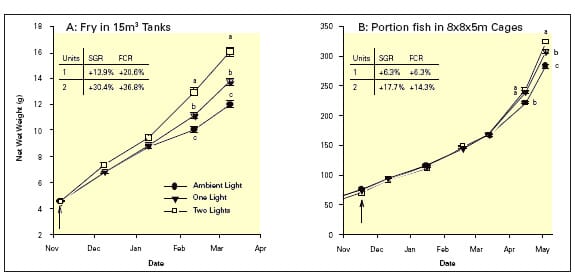
Spectral Content
Light is characteristically defined as part of the spectrum of electromagnetic radiation detectable as various colours by the human eye (visible light, λ 380-750nm). The electromagnetic radiation emitted by the sun and other sources (e.g. luminescent lamps etc.) travels as particles of energy expressed as “photons”. The amount of photon energy is inversely related to the wavelength of light, thus shorter wavelengths have greater energies, i.e. blue light (λ 450nm) has a greater energy capacity than red light (λ 700nm). Within the underwater environment, the natural light penetrating the water surface is attenuated at increasing depths by the processes of absorption and scattering by suspended and dissolved matter. The blue end of the visible spectrum penetrates seawater more efficiently, whereas in freshwater yellow-orange light penetrates further, particularly in peaty waters although this is site specific (Figure 2).
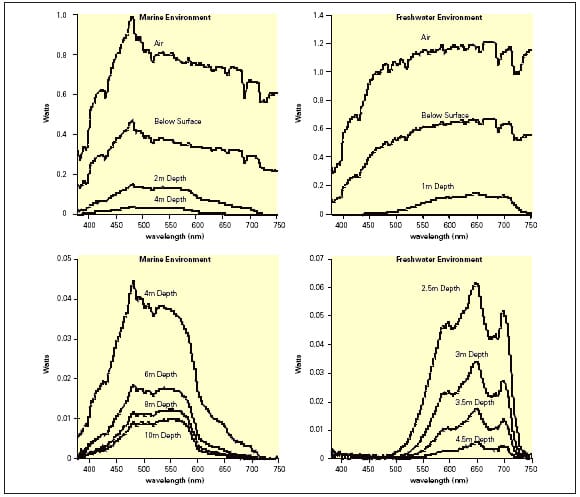
Aquaculture systems are typically lit using metal halogen bulbs, either 400W or 1000W. These have different colour temperatures which determine the main peak of the emitted light spectrum. The higher the temperature, the more the emphasis is on the blue end of the spectrum. However, significant portions of light are also released across the visible spectrum (Figure 3). The questions remain as to whether such wavelengths are biologically efficient in a given species, and is the spectral spread suitable for illuminating the particular culture environment (rearing system, water properties) or is it wasted energy? Stirling has been investigating the potential of narrow bandwidth lighting units in collaboration with lighting manufacturers. These units can be tailor made to deliver light that penetrates the water more efficiently (creating a more even light field within the system) as well as targeting the species biological sensitivities, which together will result in a greater control of maturation and growth.
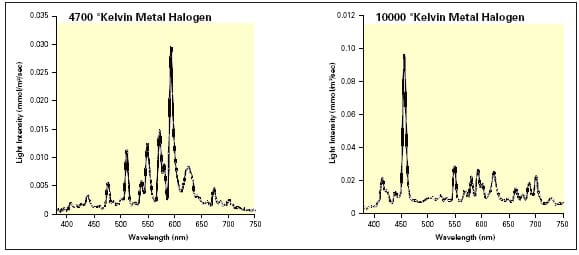
New Technologies
Technological innovations have allowed radical refinement of available lighting systems. Recent developments include Light Emitting Diodes (LED) and Cold Cathode Ray Tubes (CCL). Benefits of such systems include:
- The ability to produce narrow bandwidth light as required in comparison to standard metal halogen bulbs currently used within the industry.
- More even light distribution
- Lower power requirements which entail lower running costs.
- Increased durability
- Lower capital cost per unit
- Improved lamp life (up to 20 times the life of incandescent bulbs)
Two examples of narrow bandwidth light (cold cathode units) are under testing (Figure 4). Such novel lighting systems should improve light efficiency in aquaculture rearing systems as well as lowering running costs.
Given these considerations regarding artificial light, such technologies can be used to manipulate either reproduction and/or growth of rainbow trout.
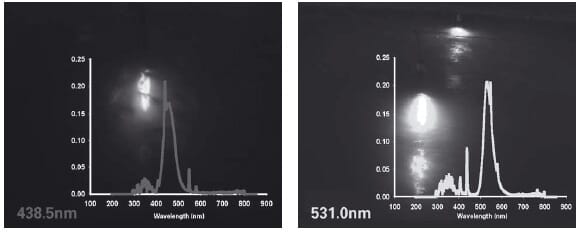
Reproduction
Photoperiod can be used to manipulate reproduction in two manners, dependent on the requirement of the farming operation. Firstly in tank based hatcheries/broodstock facilities, light can be used to alter stock spawning time to produce out-of season eggs (3,4). Secondly, light can be used to inhibit maturation prior to harvest.
Manipulating Spawning
It is now clear that seasonal changes in daylength is primarily responsible for synchronising the timing of reproduction in salmonids. Modified photoperiod regimes can be used to advance or delay the spawning period, and a year-round supply of eggs can be ensured by replacing increasing and decreasing components of the seasonally changing daylength with periods of constant long and short daylength (1). However, a major consequence of advancing spawning is that the more marked the advancement, the fewer the fish that respond (Figure 5).
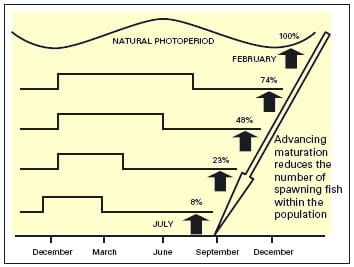
In order to compensate for this reduction in spawners, broodstock farmers may simply increase the number of individual hens within their facility. However, space constraints may prevent some producers from doing so. Recent studies carried out by the Stirling Reproduction group have investigated the importance of broodstock ration under advancing photoperiods in order to optimise spawner number. In all vertebrates, maturation only occurs when individuals have reached a certain age and size, and accumulated enough energy reserves to ensure the success of reproduction. In the case of salmonids there is a correlation between growth, adiposity and the “decision” to mature. It is also apparent that the decision to mature is taken up to one year in advance of actual spawning. In female trout, maturation typically occurs at 3 years of age, and therefore the initiation of the maturation cycle is based on growth/adiposity during the second year of life.
Our study, part of an EU funded project “Pubertiming”, presented two groups of tank-reared 1 year old female rainbow trout (hatch April 2002, expected spawn time March 2005) held under ambient photo-thermal conditions with either a LOW (normal tabulated) or HIGH (double tabulated) ration until they were 2 years old. In January 2004, the ration treatment fish were evenly divided between two tanks and exposed to either a simulated natural photoperiod (SNP) or an advancing regime (ADV; constant long-days 18L:6D until May 2004 then constant short-day 8L:16D). All fish were individually P.I.T. tagged and then fed the same ration according to manufacturer’s tables. Growth rate and spawning were monitored over the next 18 months.
The control population under SNP attained 100% maturity (Mar-Apr 2005) irrespective of ration (data not shown). In contrast, only 69% of individuals attained maturity and spawned under the ADV regime (Sept-Oct 2004) as shown in Figure 6. Significantly, of those that matured 67% were from the high ration group and only 33% from the low ration group. Thus, by doubling ration the effective spawner number was doubled. This suggests that, when attempting to alter spawning time, provision of a higher ration during the second year of life may be critical in ensuring sufficient numbers of fish spawn.
With regards to growth (data not shown) there were no significant differences in weight or growth rate between mature and immature individuals that had previously received the high ration diet under the ADV regime, although there was tendency towards a larger size for fish that attained maturity. This again supports the theory that ration and body size during the second year of life are critical in ensuring that an individual will successfully mature. These results highlight areas for consideration by broodstock farmers as a means to address shortfalls in spawner number under manipulated photoperiods.
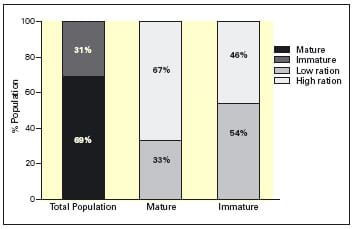
Inhibiting Maturation
The majority of table market trout farmers produce portion-size fish and, by utilising all female monosex populations and harvesting before 2 years, do not experience pre-harvest maturation problems. However, a section of the trout industry produces larger 1-5 kg fish and, because these fish are typically in the 2.5 to 3 year class, experience pre-harvest maturation problems of loss of product quality and increased mortality. Much of this large trout production is within cages where application of similar photoperiod regimes to those used successfully for Atlantic salmon, has failed to inhibit maturation. The reasons for this failure are not clear at present, although it is likely that incorrect timing of application and ineffective lighting protocols may be the key. There is also a trade-off between growth and maturity as previously discussed: an increase in maturity may be expected to be associated with fish that are pushed harder for growth. This would explain why application of light in autumn enhances growth, but has so far failed to arrest maturation in trout. However, rainbow trout maturation has been delayed by up to 4 months in open tank systems using only two months of constant light application in the autumn (2), thus the potential to delay or inhibit maturation in cages is feasible. This demonstrates that it is the timing of application that is critical. However, this option would only delay, rather than arrest, maturation.
An alternative strategy may be to advance the maturation window so much that the fish attempt to initiate maturation too early and fail. Figure 7 illustrates the key components of the reproductive cycle and decision making process and how such a photoperiod manipulation strategy could potentially inhibit maturation. Furthermore, by manipulating ration during the first few months of such a regime it may be possible to completely inhibit maturation, although this remains to be tested. The Reproduction Group at Stirling are currently exploring this avenue of research to alleviate maturation associated losses in large trout production.
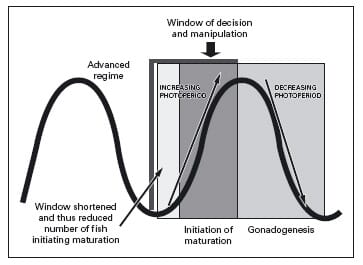
On a final note regarding maturity control, we have also been addressing the potential for improvements in triploid trout culture, particularly in saltwater as an alternative means to avoiding maturation pre-harvest in the larger fish production sector (7). Our research has made significant steps forward in the understanding of triploid physiology and their culture requirements, and we will be looking to field trial our findings in the near future at a commercial level. Performance under lighting regimes will also be assessed.
Enhancing Growth
We have previously reported the potential for enhancing growth in rainbow trout using photoperiod (3,6). Over the last few years considerable advances have been made in our knowledge of how light affects and mediates growth. Under ambient conditions, as daylength and temperature increase in spring, there is a natural surge in circulating growth promoting hormones within the fish (5). By harnessing this natural occurrence it is possible to enhance the growth of fish by applying summer photoperiods when daylengths are naturally short or decreasing (i.e. autumn), effectively tricking the body and hormonal signalling into believing it is summer and thereby boosting growth. A common question asked is when should photoperiod manipulation be applied to enhance growth in table trout production?
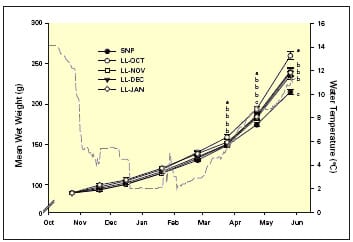
Since fish are cold blooded, their metabolic rate will be dictated by ambient water temperature and thus their response to photoperiod application will be similarly controlled. In a study exposing fish to constant light (LL) from either October, November, December or January relative to an ambient control under ambient water conditions, it was apparent that the earliest treatment with LL (i.e. October) significantly enhanced growth relative to all other treatments by the following spring (Figure 8.). There was no difference between November, December and January treatments with regards to growth, but they were all significantly larger than fish under ambient light by spring. These results strongly support the importance of applying photoperiod regimes prior to significant drops in water temperature during the autumn period. In this respect, the most effective regime would most likely be employed from the summer solstice onwards, although this will be dictated by when fish are stocked into their grow-out system.
To date much of the research and commercial trialling of photoperiod regimes has focused on tank based and cage systems. However, a significant proportion of UK trout production is centred on raceway systems. Such systems present problems when attempting to apply artificial light regimes due to their shallow depth, narrow cross-section and extensive length. Preliminary trials using four 400W metal halide floating units evenly spaced within a 50m raceway improved growth by around 5% relative to an ambient control when applied from October to March. However, as previously alluded with regards to fish light perception, the lighting fields achieved using such systems were not uniform, with light and dark patches along the length of the raceway (Figure 9). To achieve better illumination in such systems, tube and unit designs have had to change dramatically. Using CCL and LED style designs it is now possible to produce fully submersible or floating tube units up to several metres long to improve light distribution within raceways (Figure 9). The Stirling group will be looking to field trial such technologies in the near future.
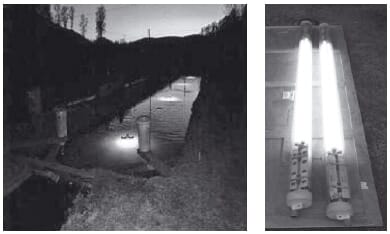
Conclusion
Although not widely utilised as yet by the trout industry, the potential for production enhancement using photoperiod is apparent and significant. With the improved understanding of the species biological requirements with regards to light, and major advances in lighting technology and associated unit cost reductions, the use of light within the industry may become more feasible.
Interested?
Experimental trials on the effects of photoperiod on reproduction and growth of trout, salmon and numerous marine species are continuing at the Institute of Aquaculture. For further details please contact Herve Migaud (hm7@stir.ac.uk) or John Taylor (jft2@stir.ac.uk).
Acknowledgements
The authors would like to thank INTRAVISION, IDEMA Aqua, BGB Engineering, NERC ROPA project (GR3/R9827) and EU FAIR (PUBERTIMING QLRT-2001-01801) for funding and technological support throughout the duration of these studies.
References
Bromage, N.R., Porter, M.J.R., Randall, C.F. (2001). The environmental regulation of maturation in farmed finfish with special reference to the role of photoperiod and melatonin. Aquaculture, 197: 63-98. Randall, C.F., Bromage, N.R., Duston, J., Symes, J., (1998). Photoperiod induced phaseshifts of the endogenous clock controlling reproduction in the rainbow trout: A circannual phase-response curve. J. Reprod. Fert., 112: 399-405. Randall, C.F., North, B., Futter, W., Porter, M., Bromage, N.R. (2001). Photoperiod effects on reproduction and growth in rainbow trout. Trout News, 32: 12-16. Taylor, J.F., North, B., Porter, M.J.R. & Bromage, N.R. (2002). Photoperiod manipulation can be used to improve growth rate and feeding efficiency in rainbow trout. Trout News, 33: 20-23. Taylor, J.F., Migaud, H., Porter, M.J.R., and Bromage, N.R. (2005). Photoperiod influences growth rate and plasma insulin-like growth factor-I levels in juvenile rainbow trout, Oncorhynchus mykiss. Gen. Comp. Endocrinol., 142: 169-185. Taylor, J.F., North, B.P., Bromage, N.R., Porter, M.J.R. & Migaud, H. (2006). Photoperiod can be used to enhance growth rate and improve feeding efficiency in farmed rainbow trout. Aquaculture, 256: 216-234. Taylor, J.F., Needham, M.P., North, B.P., Morgan, A., Thompson, K. and Migaud, H. (2007) The influence of ploidy on saltwater adaptation, acute stress response and immune function following seawater transfer in nonsmolting rainbow trout. Gen. Comp. Endocrinol. 152: 314-325.April 2008