A principal concern involves the potential genetic impacts of fertile transgenic organisms interbreeding with wild populations into which their genes may introgress. For example, risk models indicate that Trojan gene effects may occur, whereby the transgene spreads by enhanced mating advantage but the resulting offspring have reduced viability, which leads to the eventual extinction of populations (Muir and Howard 1999, 2002; Howard et al. 2004). However, there has yet to be any empirical research documenting the ability of growth hormone (GH) transgenic Atlantic salmon to breed naturally and introgress with wild populations. Moreover, there is little understanding of the role that alternative reproductive phenotypes may play in such introgression.
The breeding system of Atlantic salmon exhibits two alternative male reproductive phenotypes, large anadromous adults that have migrated to sea and returned to their natal streams, and small precocial parr that have matured in freshwater, having never been to sea. Anadromous males develop specialised secondary sexual characters to fight other males and court for access to ovipositing females, while precocial parr mature at a fraction of the size of the anadromous phenotype and use their small size and cryptic colouration to sneak fertilisations (reviewed in Fleming 1996). Both male reproductive phenotypes may form dominance hierarchies among themselves for access to spawning females through aggressive behavioural interactions. While the fertilisation success of anadromous males is typically greater than that of mature parr, reports of precocial parr fertilisation rates have ranged from 11% to 65% of the available eggs (reviewed in Fleming and Reynolds 2004). Thus, both male reproductive phenotypes can contribute substantially to the next generation and represent potential routes for the introduction of transgenes into wild populations.
The extent of transgene introgression into wild populations would depend on the fitness of transgenic individuals in the receiving environment, which may vary along a continuum featuring high fitness, leading to the fixation of the transgene, at one end and low fitness, leading to its elimination within a few generations, at the other (Muir and Howard 1999, 2002). Perhaps more commonly, however, the fitness of transgenic organisms would lie between these poles and create, for example, an outbreeding depression scenario where transgene-induced maladaptive traits pose a threat to the viability of the entire receiving population (Hedrick 2001).
This outbreeding depression scenario is representative of the concerns associated with wild salmonid populations exposed to strains that have experienced domestication selection (McGinnity et al. 2003; Tymchuk et al. 2007; Fraser et al. 2008). In Atlantic salmon, anadromous adults from aquaculture strains (farmed) exhibit atypical spawning behaviour, including reductions in aggressive displays towards other males, quivering and nest fidelity, which may contribute to observations of reduced reproductive success (Fleming 1996; Fleming et al. 2000; Weir et al. 2004). In contrast, studies exploring the relative reproductive behaviour and success of mature farmed and wild parr have found that farmed parr perform similarly to or better than wild parr (Garant et al. 2003; Weir et al. 2005). Regardless of the relative spawning success of farmed and wild males, both reproductive phenotypes have demonstrated the potential for the introgression of farmed genes into wild populations and the disruption of locally adapted phenotypic traits (Hindar et al. 2006; Garcia de Leaniz et al. 2007; Fraser et al. 2010).
Comparisons of reproductive performance between GH transgenic and nontransgenic salmonids are limited. Similar to observations with farmed adults, previous efforts have reported reduced reproductive performance in hatchery-reared transgenic relative to wild coho salmon (Oncorhynchus kisutch; Bessey et al. 2004; Fitzpatrick et al. 2011). While these results represent the expectations of a first-generation transgenic escapee scenario, GH transgenic Atlantic and coho salmon represent two species carrying two unique transgene constructs with two distinct life histories (e.g. rarely do coho salmon mature precocially as parr; Fleming 1998). For example, previous work has demonstrated differences in the onset of transgeneinduced phenotypic expression between the two species, which may have important implications for early survival (Sundstrom et al. 2004, 2005; Lohmus et al. 2010; Moreau et al. 2011). Potentially more important are the distinct differences in reproductive phenotypes that may have implications for introgression (Valosaari et al. 2008), as seen in the reproductive performance differences between anadromous and mature parr Atlantic salmon males of farmed origin (Fleming 1996; Fleming et al. 2000; Garant et al. 2003; Weir et al. 2005).
The aim of this study was to compare the breeding performance of GH transgenic and nontransgenic Atlantic salmon males of both alternative reproductive phenotypes to test for the potential of the transgene to introgress into wild populations. We conducted two separate experiments in a naturalised stream mesocosm. First, to assess the ability of first-generation, farmed transgenic males to contribute reproductively, the breeding behaviour and participation of captively reared, anadromous transgenic males (approximating farmed fish) were observed in pair-wise competitive trials with wild males, as well as alone with wild females. Second, to assess the ability of transgenic fish to contribute reproductively as precocial parr, the breeding behaviour, performance and reproductive success of captively reared, transgenic and nontransgenic precocial parr were compared in pair-wise competitive trials.
Results
Anadromous Males
The captive-reared, transgenic males were significantly larger than the wild, nontransgenic males in terms of both mass (Table 1; paired t-test; t1, 10 = 6.03, P < 0.001) and length (paired t-test; t1, 10 = 5.14, P < 0.001). Despite a clear size advantage for transgenic males, there were no differences in the frequency of overt aggressive behaviours relative to nontransgenic males (Fig. 2A; Wilcoxon signed rank test: V1, 10 = 34.20, P = 0.057). However, nontransgenic males demonstrated a competitive advantage over transgenic males in all other breeding behaviours measured. In the presence of competition, nontransgenic males spent significantly more time at the nest with the females (nest fidelity) than did transgenic males during both the prespawn and postspawn periods (Table 3). Nontransgenic males also had higher nest fidelity than transgenic males in the absence of competition. Moreover, unlike both the prespawn (LRbi X2 = 0.40, P = 1) and postspawn (LRbi X2 = 6.79, P = 0.731) periods of direct competition, there was a significant trial effect on nest fidelity (LRbi X2 = 20.56, P < 0.001) in the absence of competition, indicative of the high variation in behaviour observed. The quivering frequency of nontransgenic males was greater than that of transgenic males (LR; X2 = 41.456, P < 0.001), with no effect of competition (Fig. 2; LRpi X2 = 1.00, P = 0.606) or trial (LRpi X2 = 15.63, P = 0.111). Furthermore, nontransgenic males participated in more spawning events than transgenic males regardless of the presence or absence of competition (Fig. 3; LRpi X2 = 22.60, P < 0.001).
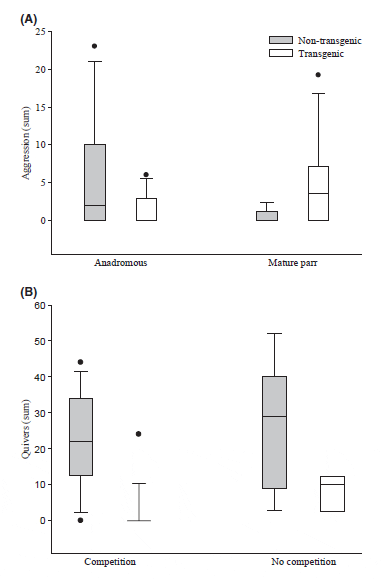
Precocious Male Parr
In trials involving 1+ nontransgenic and 0+ transgenic parr, there were no significant differences in mass (paired t-test; t1, 5 = )1.37, P = 0.231) and length (paired t-test; t1, 5 = )1.63, P = 0.163) between the two groups (Table 1). However, in trials where both parr types were age 0+, the transgenic parr were significantly larger than the nontransgenic parr in terms of both mass (paired t-test; t1, 4 = )5.325, P = 0.006) and length (paired t-test; t1, 4 = )3.47, P = 0.026). Similarly, when age is ignored and the aforementioned data are analysed collectively, the transgenic parr were significantly larger than the nontransgenic parr in terms of both mass (paired t-test; t1, 10 = )3.42, P < 0.001) and length (paired t-test; t1, 10 = )3.26, P < 0.001). There were no significant differences in behaviour between trials involving 0+ and 1+ nontransgenic parr; thus, these were combined for subsequent analyses. Transgenic parr performed more overt aggressive behaviours than nontransgenic parr (Fig. 2; Wilcoxon signed rank test; V1, 10 = 26.5, P = 0.042). However, nontransgenic parr demonstrated greater nest fidelity than transgenic parr during all the comparisons save one; nest fidelity was similar during the postspawn period of spawn A (Table 4). There were no trial effects observed on nest fidelity. Greater nest fidelity was accompanied by greater spawn participation by nontransgenic relative to transgenic parr (Fig. 3; LRbi X2 = 11.20, P < 0.001), and the levels of participation were similar across spawns (LRpi X2 = 0.13, P = 0.72).
The fertilisation success of both transgenic and nontransgenic parr was low (Table 5). Wilcoxon signed ranked tests confirmed that anadromous males dominated both transgenic (V1, 10 = 66.0, P < 0.001) and nontransgenic (V1, 10 = 66.0, P < 0.001) parr in fertilisation success across breeding trials. Furthermore, transgenic and nontransgenic parr fertilisation success did not differ significantly across trials (Wilcoxon signed rank test; V1, 10 = 16.0, P = 0.295). The overall (trial ignored) fertilisation success of nontransgenic parr, however, was significantly higher than that of transgenic parr (binomial test; X2 = 15.98, P < 0.001), and offspring fathered by nontransgenic parr were represented in more trials.
Table 3. Nest fidelity (proportion of time spent with nesting female) of anadromous growth hormone transgenic and nontransgenic Atlantic salmon males during paired competitive breeding trials. Each breeding trial included phases of competition and no competition. During competition, both the transgenic and the nontransgenic males competed directly for breeding opportunities with the female. During no competition, males had sole access to a spawning female. Data from each trial were analysed 60 min before (prespawn) and 30 min after (postspawn) each spawning event. In trials with no spawning (n = 4; all transgenic males in the absence of competition), analyses were based on observations conducted for 5-min intervals every 30 min for the duration of the phase (i.e. a total of 360 min of observation time). For statistical analyses, nest fidelity during the no competition phase was not segregated into periods.
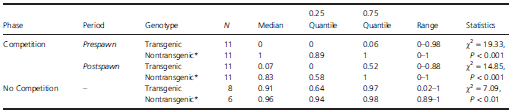
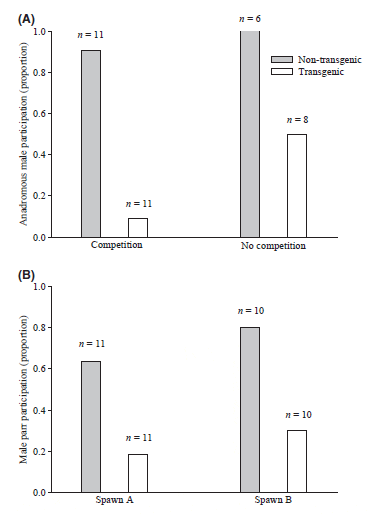
Discussion
This study provides the first empirical observation on the breeding of and potential for transgene introgression by GH transgenic male Atlantic salmon, including that of alternative reproductive phenotypes. Transgenic anadromous males (i.e. large, fighter males), reared to maturity in captivity, were behaviourally outcompeted by their wild counterparts in terms of nest fidelity, quivering frequency and spawn participation. Similarly, despite having similar rearing histories and displaying more aggression, transgenic male parr (i.e. precocially mature, sneaker males) were inferior competitors to wild-type parr in terms of nest fidelity and spawn participation. Moreover, wild-type parr had higher overall fertilisation success than transgenic parr, and their offspring were represented in more spawning trials. Although transgenic males displayed reduced breeding performance relative to nontransgenics, both male reproductive phenotypes demonstrated the ability to participate in natural spawning events and thus have the potential to contribute genes to subsequent generations.
The reduced reproductive performance of captively reared, anadromous transgenic males relative to wild males parallels the results of similar studies comparing captively reared salmon to wild salmon. Varying degrees of exposure to captive environments and domestication selection have been shown to affect the breeding behaviour and success of adult salmonids negatively (Fleming and Gross 1993; Fleming et al. 1997; Berejikian et al. 2001a; Weir et al. 2004). Moreover, Bessey et al. (2004) observed that wild-exposed coho salmon males outcompeted captively reared transgenic males in terms of spawn participation, courtship and aggressive behaviours. Bessey et al. (2004) also observed that when transgenic and nontransgenic males were both reared in the laboratory, performance was poor irrespective of transgenesis (see also Fitzpatrick et al. 2011). Thus, the captive rearing environment appears to diminish the competitive and reproductive performance of the anadromous salmonid phenotype, irrespective of genetic background (Berejikian et al. 1997, 2001a,b). The current study can, therefore, not eliminate the possibility that the poor performance of the anadromous transgenic males has more to do with rearing environment than transgenesis because these variables were confounded. Nevertheless, comparisons of captively reared transgenic and wild andromous males mimic the environmental differences that represent an initial transgenic escapee invasion scenario and are thus valuable for predicting the probability of first-generation intraspecific hybridisation.
Table 4. Nest fidelity (proportion of time spent with nesting female) during paired competitive breeding trials of mature male parr that were growth hormone transgenic and nontransgenic. The first (A) and second (B) spawns from each trial were analysed for the period 52.5–12.5 min before the spawn (prespawn), 12.5 min on either side of the spawn (spawn), and 12.5–22.5 after the spawn (postspawn).
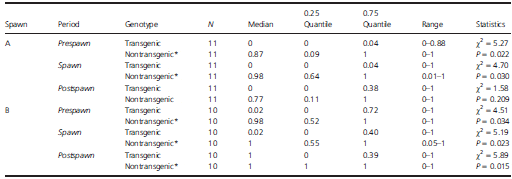
Table 5. The fertilisation success (proportion of eggs fertilised) of wild anadromous males and growth hormone transgenic and nontransgenic mature male parr during 11 pair-wise competitive breeding trials. Representation indicates the number of trials where successful fertilisation was observed by a male type.
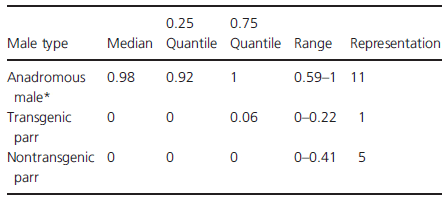
Reproductively isolated populations are predicted to genetically diverge because of adaptive and/or nonadaptive evolutionary pressures, such as selection to environmental variation, genetic drift, gene flow and chance mutations (Frankham et al. 2002; Allendorf and Luikart 2007; Garcia de Leaniz et al. 2007; Carlson and Seamons 2008). This evolutionary theory provides some perspective on two elements of the current study. First, the captively reared, anadromous transgenic males did not have an identical genetic background to the wild anadromous males with which they were compared. Specifically, the genetic background of the transgenic males consisted of two wild populations, one of which was the same as that of the wild males. Thus, in addition to captive rearing, intraspecific population differences may also have contributed to observations of reduced reproductive performance in transgenic relative to nontransgenic males. Second, evolutionary divergence among wild Atlantic salmon populations can potentially influence their relative reproductive performance when competing against transgenic invaders (Devlin et al. 2006; Kapuscinski et al. 2007; Hutchings and Fraser 2008). This study correctly mimics a likely invasion scenario, where the genetic background of the transgenic population differs from that of the wild population. However, contextualising these results with the general concerns of GH transgene introgression into wild populations must be performed with caution. It remains uncertain how the reproductive performance of this GH transgenic population would compare with other wild populations. Similarly, it is uncertain how the reproductive performance of this wild population would compare with other GH transgenic populations.
Previous studies comparing the reproductive behaviour and success of farmed and wild-type mature male parr have suggested that this alternative male reproductive phenotype may facilitate the interbreeding and introgression of farmed genes into wild populations (Garant et al. 2003; Weir et al. 2005). This rationale is based on observations of equal or greater breeding performance among farmed parr relative to wild-type parr coupled with the fact that maturation prior to anadromy increases the probability of survival to maturity and reduces generation time. In the current study, we found that the breeding behaviour and success of transgenic parr was inferior to that of wild-type parr, despite transgenic parr displaying more aggression. Moreover, transgenic parr sired fewer eggs than nontransgenic parr. When the data were paired by trial, however, no differences were observed in fertilisation success between the two groups, which may be due largely to a number of spawns where there was no parr contribution and the associated low statistical power. Interpretations based on the entirety of the behavioural and fertilisation findings suggest that the nontransgenic parr marginally outcompeted transgenic parr during spawning. Nevertheless, transgenic parr demonstrated a behavioural interest in spawning and contributed gametes to the next generation. Thus, the alternative male reproductive phenotype of early maturation in Atlantic salmon may facilitate the introgression of transgenes into wild populations in a similar manner to that observed with farmed strains.
In an effort to limit size differences between transgenic and nontransgenic parr during the paired behavioural trials, age differences existed between competing parr in some of the trials. There was no significant difference in transgenic performance, whether competing with 0+ (n = 5) or 1+ (n = 6) nontransgenic parr, although we acknowledge the statistical limitations associated with the low sample sizes. Moreover, despite holding a significant body size advantage, irrespective of nontransgenic parr age, and exhibiting increased overt aggressive behaviour, the reproductive success of transgenic parr was less than that of nontransgenic parr. While there is evidence both for (Thomaz et al. 1997; Koseki and Maekawa 2000) and against (Jones and Hutchings 2001, 2002) parr body size influencing spawning success, it has been suggested that large body size may be a stronger predictor of dominance under scenarios with few competing parr (Hutchings and Myers 1994; Jones and Hutchings 2001). However, in the present study, the breeding performance of transgenic parr appears to be inferior independent of size.
The reduced breeding performance of transgenic parr may be due, in part, to behavioural changes associated with GH transgenesis. Juvenile salmonids have shown distinct shifts in behavioural phenotypes in response to GH transgenesis, including increased foraging-induced aggression and reduced antipredator behaviour (Abrahams and Sutterlin 1999; Sundstrom et al. 2003, 2004). The reduced nest fidelity and spawn participation by mature transgenic parr relative to nontransgenic parr may be driven by transgene-induced hormonal changes. Gonadotropinreleasing hormone (GnRH) is thought to increase the expression of reproductive behaviours in many species (Maney et al. 1997; Yamamoto et al. 1997; Johnson et al. 2007; Munakata and Kobayashi 2010), including salmonids (Berejikian et al. 2003). For example, studies with the dwarf gourami (Colisa lalia) have indicated that male nest building behaviour is reduced when GnRH function is disrupted (Yamamoto et al. 1997; Munakata and Kobayashi 2010). Moreover, there is an existent, but poorly understood, association between the GH-IGF-I axis and the GnRH-gonadotropin-sex steroid axis (Holloway and Leatherland 1997a,b; Mercure et al. 2001; Bjornsson et al. 2002). Thus, GH transgenesis may influence the interactions between these two hormonal axes such that the breeding behaviour of mature male parr is negatively affected. However, empirical investigations are required to explore the effects of GH on reproductive hormones and behaviour.
A common method for conducting environmental risk assessments involves the use of quantitative models that estimate a defined measure of risk. For genetically modified organisms, the prospect of gene flow from transgenic escapees into wild populations is a key issue because of the potential influences the transgene may have on fitness. In response, models have been developed to estimate the fitness outcome of transgene introgression into wild populations (Muir and Howard 1999, 2001; Aikio et al. 2008; Valosaari et al. 2008; Ahrens and Devlin 2010). Frequently, the model parameters consist of empirical measurements of fitness-related life history traits such as growth, survival and reproductive probabilities, age at sexual maturity, female fecundity and male fertility (Muir and Howard 2002). The current study provides data on the relative breeding success of male salmon that are applicable to such predictive quantitative models. Specifically, we contribute to observations indicating captivereared GH transgenic and farmed adult male salmon have a mating disadvantage relative to wild individuals, a gene flow scenario indicative of an initial invasion. Moreover, captive-reared nontransgenic precocial male parr demonstrated a modest mating advantage over transgenic individuals, a gene flow scenario comparable to subsequent generations following an invasion. Similar to the Japanese medaka (Oryzias latipes) work of Pennington et al. (2010), these findings are inconsistent with the assumption of a transgenic male mating advantage used in previous quantitative models (Hedrick 2001; Aikio et al. 2008; Valosaari et al. 2008), but see Howard et al. (2004), and emphasise the importance of basing parameter values on empirical data.
The present study, however, only provides an estimate of breeding success under a single set of physical and demographic environmental conditions consisting of paired males competing for single females. In the wild, male salmon will typically have access to multiple females simultaneously and have to contend with multiple competitors (Fleming 1996; Fleming and Reynolds 2004). Moreover, should transgenic animals get exposure to the wild environment prior to breeding (i.e. escape prior maturation), this may well alter their reproductive performance in a similar way, but opposite, to the effects captive rearing has on wild fish (e.g. Berejikian et al. 1997, 2001a; Bessey et al. 2004). As pointed out by Devlin et al. (2006), there are limitations and difficulties associated with collecting the breadth of empirical data required to accurately represent the full range of genotype by environment interactions affecting fitness-related life history traits in the wild. The findings of this study are valuable with respect to a first-generation invasion scenario; but beyond that, reproductive performance is difficult to predict and is, therefore, an unavoidable source of epistemic uncertainty for both quantitative and qualitative invasion models. Further work is thus required to compare the breeding performance of transgenic and nontransgenic salmon in a range of ecologically relevant scenarios.
![]() |
Further ReadingYou can view the full report by clicking here. |
May 2013