Biology of the Hard Clam
Hard clams of the genus Mercenaria are found from the Gulf of St. Lawrence to the Gulf of Mexico and have been introduced to other areas of the United States, notably the coasts of California and Washington. They also have been introduced to Puerto Rico and Great Britain. There are two species, Mercenaria mercenaria and M. campechiensis. M. mercenaria is distributed primarily in the more northerly latitudes, while M. campechiensis is the more southerly species. Both species are found on the west coast of Florida and in Georgia, South Carolina and North Carolina, and some hybridization between the two may occur. A subspecies, M. c. texana, is found in the western Gulf of Mexico. This fact sheet refers specifically to M. mercenaria because it is the major cultured species, although some of the generalizations may apply to all hard clams.
The hard clam is rarely found where salinities average less than 20 parts per thousand (ppt). Hard clams occupy intertidal and subtidal habitats, where they burrow into the substrate to various depths (normally less than 20 cm or 8 inches). They are found in a variety of substrates, including sand, mud, shell and mixtures of these materials. Clams are filter feeders and remove food particles, primarily small phytoplankton (single-celled algae), from the water. An adult clam filters an average of 7 to 8 liters (about 2 gallons) per hour.
Hard clams usually reach sexual maturity at a shell length (SL) of about 35 mm (about 1.4 inch) (Fig. 1). The sexes are separate but are externally indistinguishable. Clams are protandric, maturing as males at an early age and changing sex in subsequent years to spawn as females. When stimulated by appropriate environmental conditions (normally high water temperatures), clams release gametes (sperm or eggs) into the water. The presence of gametes in the water stimulates other clams in the immediate vicinity to begin spawning. Fertilization occurs in the water column. Clams usually spawn intermittently from May through October. A female clam can release several million eggs in a single spawning, but relatively few survive the larval period to become juvenile clams in the wild.
Fertilized eggs (zygotes) undergo rapid cell division and within 12 hours develop into free-swimming trochophore larvae. Within another 12 hours, bivalve shells have formed and the larvae are in the veliger, or straight-hinge, stage. Veligers are often referred to as “D” larvae because their shape resembles a capital letter “D.” Before the veliger stage the larva is sustained by lipids stored in the egg and by dissolved organic matter (DOM) absorbed from the surrounding water. The veliger feeds on small phytoplankton, bacteria and DOM. The ciliated velum, which gives the veliger its name, is used for both locomotion and feeding. The length of the larval period depends largely on temperature and food supply. After 7 to 21 days the veliger larva develops a foot and is called a pediveliger (Fig. 1). This stage is brief. The clam soon loses its swimming organ (velum) and develops siphons. This is referred to as settlement, and newly settled clams may be called “set” or “post-set.” Postset clams assume the sedentary lifestyle of the adult. The term post-set is informal and usually refers to clams that have completed the larval stage but are still housed in a hatchery.
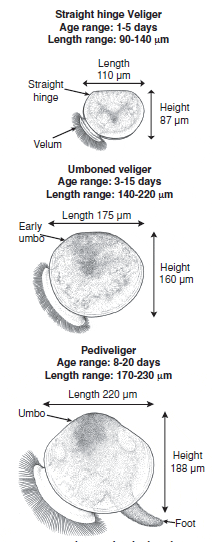
Juvenile clams less than 35 mm (about 1.4 inches) SL are called seed. As seed grow to market size they are classified into commercial categories, which are arbitrary classifications that may vary from state to state and dealer to dealer. In most states the smallest legal size for wild-caught clams is a littleneck, usually defined as 1 inch (25 mm) thick. This generally corresponds to a shell length of 45 to 50 mm (1 to 2 inches). Slightly larger clams (1.25 inches or 30 mm thick, 50 to 60 mm or 2 to 2.5 inches SL) are referred to as topnecks. Cherrystones are approximately 1.25 to 1.5 inches (32 to 38 mm) thick and 65 to 79 mm (2.5 to 3 inches) long. Anything larger than that is a chowder clam. Littlenecks command the highest price of the commercial categories. In some states maricultured clams may be sold at smaller sizes and some companies have created their own names for these size classes. In the southern U.S. wild clams grow to littleneck size in 2 to 4 years, while farm-raised clams may reach this size in about 1 to 2 years.
Hatchery Culture
There are five main processes in the hatchery culture of the hard clam: 1) maintenance and conditioning of broodstock; 2) spawning; 3) larval culture; 4) post-set culture; and 5) food production (algal culture). These activities are supported by a water distribution and treatment system, air distribution system, freshwater washdown facilities, supplemental lighting for algal culture, and test equipment and instrumentation.
Maintenance and Conditioning of Broodstock
Adult clams are brought into the hatchery for “conditioning” several weeks before spawning. Conditioning is the process of inducing gametogenesis, or the ripening of gonads, to make clams ready for spawning. In the southern U.S. clams become naturally ripe in the spring and remain intermittently ripe into the fall. To get them to ripen at any other time of year, one must simulate early spring conditions of cool temperature (18 to 20 °C) and ample food. Clams will usually condition in 2 to 8 weeks, but this varies with the time of year and the physiological condition of the clams. A clam that is already ripe can be maintained in this condition for a long period (up to 6 months) by keeping it in cool water (18 to 20 °C) and providing abundant food. Partially ripe clams will be ready to spawn in 2 to 4 weeks under these same conditions. It is much more difficult to condition clams that have recently spawned. So for year-round operation it may be advisable to collect naturally ripe clams in early spring and keep them in conditioning tanks to maintain a population of readily spawnable individuals. Naturally ripe clams also can be collected in the fall, although fewer wild clams are ripe at this time than in the spring. The general requirements for maintaining and conditioning broodstock are listed in Table 1.
Spawning
When clams spawn they release eggs or sperm into the water column, where fertilization takes place. Spawning is induced by alternately heating and cooling the clams in a water bath. Sperm or eggs from a sacrificed clam also can be used to stimulate spawning. If controlled breeding is desired, the clams are spawned in individual beakers so fertilization can be controlled. If the objective is simply to produce larvae and the parentage is unimportant, it is easier to “mass spawn” the clams in a common container. Sperm from spawning males will stimulate other clams to spawn also and the eggs will be fertilized in the common spawning container.
Larviculture
Zygotes (fertilized eggs) are maintained in clean, filtered seawater at relatively high densities (30 or more per milliliter of culture water) for 24 hours or until the veliger stage is reached. At that point the larvae are thinned to 5 to 10 per ml and given food (algae). The veliger stage lasts 7 to 21 days, depending largely on temperature and food quality (Table 2). During this time the larvae grow from 100 ?m to 180 to 250 ?m and are gradually thinned to a final density of 1 per ml. Larvae are kept in static cultures, the water is changed frequently (daily if possible), and food is added daily. Some hatcheries aerate larval cultures, but this is not usually necessary. Water for larval culture must be filtered (1 to 10 ?m) to remove silt and native plankton. Depending on the quality of the seawater, it may also need to be treated with charcoal to remove dissolved organics and sterilized (usually with ultraviolet light) to kill bacteria.
General Culture Requirements for Maintaining and Conditioning Broodstock Clams in Closed System Raceways.
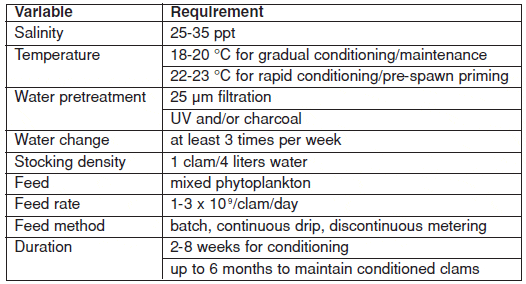
Larval culture containers may be made of fiberglass or plastic and are usually no more than 1 meter deep (3 feet). Container size is dictated by the amount of larval production desired. Plastic buckets (20-liter, 5-gallon) can be used to grow small quantities of larvae (20,000). Large hatcheries have fiberglass tanks that may hold several thousand liters of water and millions of larvae. Containers can be flat-bottomed or conical-shaped. Conical bottoms facilitate draining and cleaning. Tanks may have valved drains at the bottom or may be drained with siphon hoses. When the water is drained from the containers, the larvae are captured on a fine-mesh nylon or polyester screen.
Toward the end of the larval period, usually at a size of 200 to 250 ?m, the larvae begin the metamorphosis to the juvenile form. The first indication of this metamorphosis is the appearance of a foot. This stage is called “pediveliger” because the larva has both a velum and a foot (Fig. 1). The pediveliger stage is fairly short; within a few days the velum disappears entirely and a siphon is formed. This metamorphosis is known as “setting” and the young clams are referred to as “set” or “post-set.”
Post-set Culture
Pediveligers are often removed from the larval culture system and kept in a separate post-set culture system until metamorphosis is completed. This improves survival because pediveligers, although still capable of swimming, spend most of the time crawling on the bottom of the tanks where waste products and dead larvae are concentrated. Post-set clams may be cultured in a variety of systems. Many hatcheries use shallow trays in a gentle flow of seawater, augmented with cultured algae. These trays can be stacked in tiers to save floor space. An alternative is a recirculating culture system with downwellers and/or upwellers. This system is easier to clean than a tray system, makes it easier to control water quality and feeding rations, and supports a large number of clams in a small space (Table 3).
Downwellers (also known as “silos”) are open-ended cylinders, usually constructed of plastic pipe, suspended in a reservoir. The bottom of the cylinder is covered with a fine mesh that supports the clams (Fig. 2). Before they can be moved to silos, the clams should be large enough to stay on a 150-?m mesh. Smaller mesh will clog too readily, obstructing the flow of water. Water (and food) is circulated through the silos with airlifts (Fig. 3). In a downweller, the airlift is positioned outside the cylinder and moves water from the reservoir into the silo. Downwellers are used for early post-set that might be sucked up the airlift. When the post-set reach a size of about 0.5 mm SL, the silos can be converted to upwellers by moving the airlift to the inside of the cylinder, drawing water up through the clams. The silos are suspended in a reservoir (a container accommodating one or more silos). The reservoir volume should provide at least 0.5 ml water for each clam. Food is added to the reservoir either in batches or by continuous delivery. All the water in the reservoir is changed regularly— daily if possible, but at least three times a week.
Post-set clams are usually maintained in a hatchery until they are at least 1 mm or can be retained on a 710-?m mesh. At that point, the clams may be transferred to a nursery culture system.
General Culture Requirements for Larval Hard Clams in Closed System Tanks.
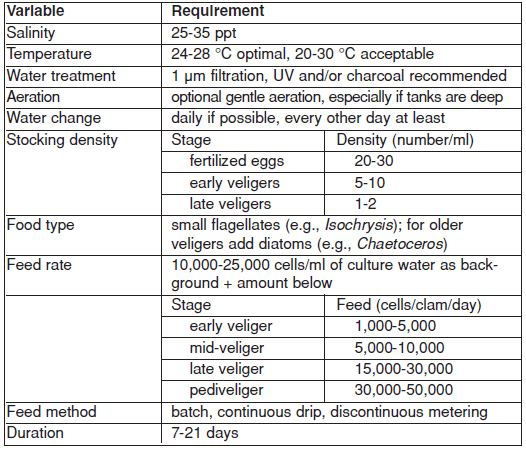
Food Production
Broodstock, larvae and post-set are fed phytoplankton produced in the hatchery. There are three basic methods of algal production: the “Wells-Glancy” method, the “brown water method,” and the “Milford” method. The Wells- Glancy method and the brown water method are relatively low-tech and inexpensive, but are not as reliable as the Milford method for producing consistently high-quality food. They are most often used in seasonally operated, low-budget hatcheries or as a supplement to the Milford method.
The Wells-Glancy and brown water methods both rely on the native phytoplankton available in the seawater supply. The water is filtered (5 to 15 ?m) to remove zooplankton and used immediately as culture water for larvae (the brown water method) or allowed to bloom for 24 to 48 hours before being used. Fertilizer can be added to encourage a denser bloom. Shallow tanks are usually used for this method so that light can penetrate. Tanks should be aerated gently. This type of culture is usually done in a greenhouse or solarium, although artificial lighting may also be used.
General Culture Requirements for Post-set Hard Clams Raised in Downwellers Housed in Reservoir Tanks. The System is Converted to Upwellers as Clams grow to 0.5 mm SL.
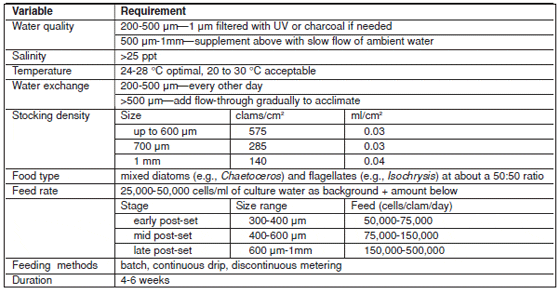
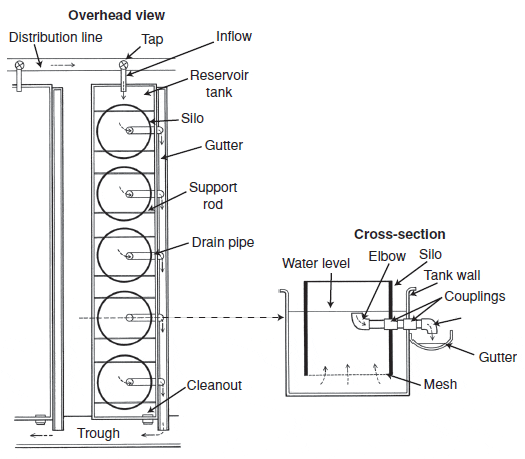
The Milford method of algal culture is a sequential process in which a single species of phytoplankton is grown in batch cultures. Phytoplankton for culture can be obtained by isolating native species, but they are usually purchased from another hatchery or from a laboratory that specializes in isolating and producing phytoplankton clones. Most hatcheries grow two or more different species of phytoplankton to provide the varied rations required by clam larvae, post-set and broodstock.
The quantity of phytoplankton needed depends on the seed production goals of the hatchery. Algal consumption rates for different size clams are presented in Table 4. Fifty broodstock (a minimum for a spawning) will require about 1.5 x 1011 algal cells each day; that will be 30 to 50 liters of dense algal culture containing 5 x 106 cells per ml. One million veliger larvae will consume 1 x 109 cells each day (1 liter); a similar number of setting size larvae will consume 50 times as much. A million post-set clams will require three to four times as much algae as the broodstock (200 liters per day). Algal culture is probably the most time-consuming and challenging element of the hatchery operation, but it is essential to its success.
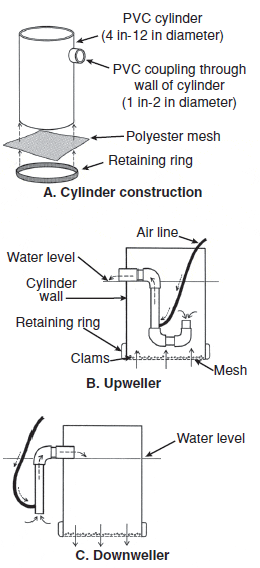
Nursery Culture
When post-set clams reach a size of 1 mm SL (retained on a 710-?m screen) they can be transferred to a nursery system. The purpose of a nursery is to provide a protected environment for small seed until they reach a size (8 to 10 mm) suitable for field grow-out. Studies have shown that survival in field grow-out increases with seed size. However, it is difficult to keep clams larger than 10 mm in a nursery because of space and water flow limitations. Some Florida growers plant their seed at smaller sizes (6 to 7 mm), but survival varies.
There are many types of nursery systems. These can be roughly divided into land-based and field-based systems. Common land-based systems are raceways and upwelling systems. Field-based systems use a variety of on-bottom and off-bottom containers, floating rafts, and floating upwellers. Also included under field-based systems are nurseries located in protected areas such as impoundments and ponds.
Field-based systems are relatively inexpensive to construct and operate but have high maintenance requirements, offer limited protection from predators, may be subject to environmental damage, and have unreliable production. Land-based systems are more expensive to construct and operate but provide almost complete predator control, ease of access, and near-optimal conditions for the growth and survival of seed clams.
Land-based Systems
A land-based nursery is a semi-controlled environment for culturing juvenile clams. Water is usually not treated except for gross (200 ?m) filtration to reduce the influx of fouling organisms (e.g., barnacles and oysters), and the natural food supply is not supplemented. However, predators are excluded, fouling organisms are controlled, and conditions necessary for rapid growth are provided. Clams can be introduced into a nursery at a size of 1 mm or larger and are usually grown to a size of at least 8 mm before they are transferred to field grow-out. Clams can grow as much as 2 mm per month. Therefore, it will take 3 to 4 months (at least) for a 1-mm clam to reach 8 mm, and another month for each 2 mm of growth beyond that size.
The two basic types of onshore or land-based nursery systems are raceways and upwelling systems. Both are energy-intensive, requiring the continuous pumping of large volumes of highquality estuarine water. Both require considerable capital investment for waterfront property and infrastructure. Both are labor-intensive, requiring daily cleaning and frequent monitoring of the seed. Despite these drawbacks, onshore nurseries are popular because they promote rapid growth and have high seed survival rates.
Estimated Consumption Rates and Recommended Feeding Rates for Different Life Stages of Hard Clams fed a Diet of Isochrysis galbana.
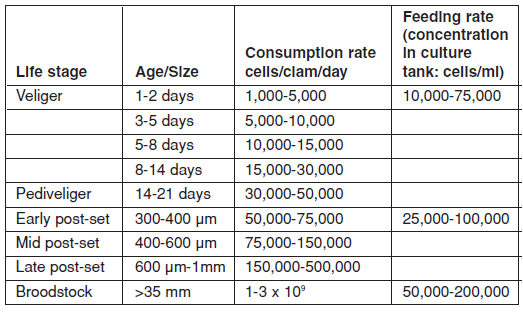
Raceways
Raceways are shallow, rectangular trays that may be stacked in tiers. Water is introduced at one end, flows over the seed clams, and exits at the other end through a drain. Ten to 20 liters of water per minute should be supplied for each liter of large seed clams (8 mm). Although raceways produce rapid growth with good survival, they are not well-suited to areas where the water supply has a high load of fine silt. Silt settles in the raceways and creates labor-intensive maintenance problems. If not cleaned daily, small seed clams suffocate in this fine silt. Cleaning is particularly difficult if the seed are very small (less than 4 mm). Another drawback with raceways is that clams near the drain end receive only water that has already passed over all the other seed and is largely devoid of useable food. Thus, the seed must be rotated in the raceway to achieve uniform growth. Finally, raceways cannot support large biomasses of seed. A typical raceway can support about 0.2 L of large seed per 0.1 m2 (1 ft2). This is about 1,400 seed at 8 mm SL or 700 at 10 to 12 mm SL. Raceways probably should not be used for seed smaller than 4 mm SL because of the cleaning difficulties. Seed need about 2 months to grow from 4 to 8 mm in raceways.
Upwelling Systems
Upwelling systems are probably the most widely used land-based nursery systems. Although they, too, have high capital costs and energy expense, upwellers promote the rapid, uniform growth of seed; they are easier to maintain than raceways and make more efficient use of space. An upwelling system consists of a reservoir (usually rectangular and about 2 feet deep) that contains several culture units (Fig. 3). Each culture unit (“silo”) has a screen bottom that supports the seed clams. The silos are placed so that their sides project above the water level and the bottom screen is several centimeters above the reservoir bottom. A drainpipe near the top of the silo extends through the silo wall and the reservoir wall and empties into an external common drain. Water is pumped into the reservoir, rises up through the screens and the seed clams, and exits out the drains near the top. The vertical water flow supports much larger biomasses of clams than the horizontal raceway flow. Each 0.1 m2 of upweller screen can support 1.0 to 1.5 liters of planting size seed (7,000 to 10,000 seed at 8 mm SL). The water flow requirements for upwellers are similar to those for raceways. Each liter of large (7-mm) seed requires a flow of 10 to 20 liters per minute; very small seed (less than 3 mm) should receive ten times that much (Table 5).
Field-based Systems
Field-based nurseries are not as reliable as land-based systems, but they require less capital investment and are less expensive to operate. They do not protect clams from predators as well as land-based systems and are more susceptible to environmental damage (e.g., from storms). They are less accessible and consequently more difficult to maintain, but the maintenance is done less frequently. The water flow (and thus food supply) to the seed may be variable, which results in less uniform growth. However, some floating systems rival land-based nurseries in production capability and are relatively inexpensive to build and operate.
Floating Upwelling Systems
Three floating upwelling systems have been used to some extent in the southern U.S. Two of these are powered by airlifts and one is tidal-powered. Operation and maintenance is similar to that for a land-based system. Floating upwellers have been used with very small seed (1 mm), but their success depends on proper site selection.
Both airlift systems are designed for use in protected waters such as saltwater ponds or impoundments. They can also be moored to a dock in a tidal creek if they will not be exposed to heavy wave action. One airlift system uses a raft of 4-inch PVC pipe to support the silos and an air manifold. The manifold is pressurized with a small air blower. Silos are suspended within the raft and water is circulated through the silos with airlifts (Fig. 4). The second airlift system consists of a reservoir (tank) that is floated in a pond or adjacent to a dock. Water is pumped into the reservoir with a large airlift or with a submersible pump and flows out the silo drains as in a land-based system.
A tidal-powered upwelling nursery consists of a raft with a scoop on one end and an interior chamber where upwellers are housed (Fig. 5). The raft is tethered from the scoop end so that it swings to point the scoop into the current. Water is directed by the scoop into the silo chamber and flows out through sideports at the water level. In pilot testing, this system appeared to foster more rapid growth than a land-based nursery. It offers the obvious advantage of low operating costs (maintenance only), but is vulnerable to storm damage.
Bottom Culture
Various bottom culture nursery systems are in use in the southern U.S. These include trays supported off the bottom on racks in subtidal areas, trays planted on or just above the bottom in intertidal and shallow subtidal areas, and “soft” bags or pens. All bottom culture systems require frequent maintenance to remove silt, fouling organisms and predators.
The subtidal rack and tray system must be deployed in deep water so it will not be a navigation hazard. Therefore, a large boat is needed to deploy, maintain and harvest the trays. Trays are made of wood frames with screening top and bottom. Several trays are supported on one rack in a tiered arrangement, with space between the trays for water circulation. A rack and tray system is laborintensive to maintain but, if properly sited, promotes good growth and survival even for very small seed (1 mm and up). Small seed (1 to 2 mm) can be planted at very high densities (50,000 to 150,000/m2, about 5,000 to 15,000/ft2) initially, but must be thinned as they grow. The fine mesh that must be used to retain very small seed has to be cleaned weekly to remove fouling organisms and silt.
Flow Requirements and Stocking Densities for Seed Clams in an Upwelling Nursery.
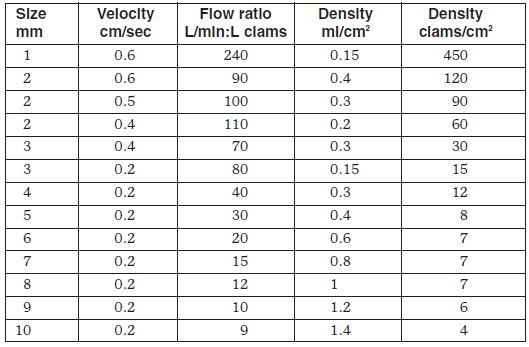
Smaller trays can be deployed in intertidal or shallow subtidal areas if substrate is firm (sand or shell). The trays are buried so that their tops are level with the surrounding substrate; then they are filled with sand and covered with screening (6-mm or 0.25-inch openings). If the trays are buried too deep they may be covered over by shifting sand or silt. If they are not buried deep enough, they may create scouring currents that will wash out the tray. Banks that have sandy substrate are often subject to strong currents that may wash substrate out of the trays. Screens must be checked often and any silt removed from the top screen. Site selection is important because heavy silt can kill clams. These trays are probably not suitable for very small seed (less than 2 mm).
Trays or polyethylene mesh bags may be placed on racks in intertidal or shallow subtidal areas. Racks may be constructed of wood, rebar or PVC. Trays with solid bottoms are filled with sand or similar substrate. Bags will not retain substrate and will work only in well-protected sites with no waves or strong currents; bags and are not wellsuited to seed less than 4 mm.
“Soft” bags are used extensively in Florida for nursery culture and have been tried farther north with mixed success. In Florida, cages with several tiers of bags have also been tried. Predation of small seed clams (less than 7 mm SL) by crabs and rays has been a problem in “soft” bag nurseries. The bags are made of woven polyester in an appropriate mesh size to contain the seed. Bags are anchored to the bottom with a weighted rope, rebar or stakes. A float inside the bag holds the upper side above the seed. In very silty areas, the float is often not adequate to prevent silt from collecting on the top of the bag.
A modification of the “soft” bag is the “soft pen.” These pens are constructed of the same soft, woven material but have a rigid frame that supports the upper edge 25 to 36 cm (12 to 18 inches) above the substrate. The frame is made of PVC pipe and placed on the inside or outside of the mesh. A recent development is the use of a vinyl covered wire (14-gauge, 0.5-inch openings) skirt to support the soft pen. The wire fencing helps keep out predators. Pens have removable tops made of the same soft mesh or of lighter weight plastic netting. The lid must have a mesh size small enough to exclude most crabs and it must fit securely over the edge of the pen so that there is no gap where crabs can enter. These systems are not suitable for small seed (less than 4 mm) because the mesh size required to retain such little seed would foul rapidly and restrict water flow.
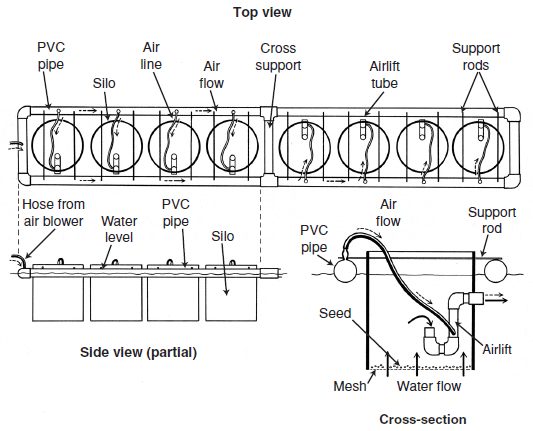
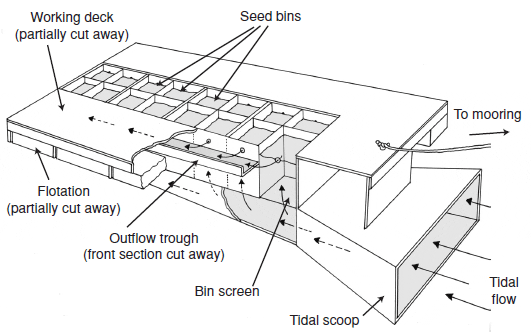
Pond Culture
Ponds and impoundments can be used as nurseries to some advantage. They protect clams somewhat from environmental disturbances and predators and are usually more accessible than field sites, while being less expensive to operate than land-based nurseries. However, it is very expensive to construct a pond so this method is probably an option only where a pond already exists.
With pond culture the natural food supply can be enhanced by managing for algal blooms. However, pond management is complex and poorly understood, particularly as it relates to the requirements of bivalve culture. In addition to the food supply, the pond manager must consider many other factors that will affect clam growth and survival, such as dissolved oxygen, pH, temperature and waste products.
Various sizes of seed have been planted in a variety of containment systems, including airlift-driven upwellers, trays and bags supported on racks, trays and bags placed on the bottom, and pond-side upwellers to which water is pumped. Seed have also been planted on window screening placed directly on the pond bottom. In pond culture, containers mainly facilitate the handling and harvesting of seed and are less important for predator control, as predators are physically excluded from the pond.