Deforestation, industrial agriculture, harvest, pollution, and urbanization are among the anthropogenic factors that have produced the most profound changes (Foley et al. 2005).
In contrast to the attention that ecological responses to human disturbance have received, evolutionary consequences of these large-scale anthropogenic changes have been relatively neglected (Tseng 2007).
The nature and magnitude of these evolutionary responses will be determined by interactions between the characteristics of the ecological changes and the biology and life history of the affected species.
Humanity already captures and uses more than half of the available fresh water on a global basis (Postel et al. 1996), largely through diversions and impoundments to rivers (Vitousek et al. 1997). Major dams typically remain in place for decades to centuries, producing long-lasting changes to natural ecosystems. These effects are pervasive: approximately 40 000 'large' (> 15 m tall) dams are in place worldwide (ICOLD 1998), and the vast majority of major rivers are impounded (McCully 2001). These impoundments have a particularly dramatic effect on the distribution, abundance, and life histories of migratory aquatic species (Merritt & Wohl 2002).
Among the major rivers most profoundly affected by dams is the Columbia River in the northwestern USA, which drains an area the size of France. By 1900, hundreds of small dams had been built within the basin to transport lumber (Sedell & Luchessa 1982) and supply water for municipal, industrial, irrigation, and livestock uses. Beginning in late 1800s, hydroelectric dams were constructed on larger tributaries.
The Great Depression witnessed construction of federal hydropower projects of unprecedented size at Bonneville Dam and Grand Coulee Dam, to provide energy to fuel development of the interior Columbia River basin.
Subsequently, nine more large dams were built between these structures on the Columbia, as well as four on the lower Snake River, the Columbia's major tributary. Over 100 large hydroelectric and multipurpose dams currently exist within the basin (NRC 1996).
Historically a Producer of Pacific Salmon
The Columbia River basin was, historically, also a major producer of Pacific salmon (Oncorhynchus spp.), supporting an estimated 8–10 million adults per year prior to European contact (NRC 1996). The rich diversity of salmon populations within the basin was forged during repeated Pleistocene episodes of glacial expansion and retreat and by more recent Quaternary events.
A great deal has been written about, and a large amount of money spent on, the effects of the Columbia River hydropower system on Pacific salmon (e.g. NRC 1996; Dietrich 2003; Williams 2006).
Mainstem dams have transformed a free-flowing river into a series of long lakes. Impassable dams preclude access of anadromous fish to 45% of their historical habitat area within the basin, and those dams with passage facilities present special challenges for both juvenile and adult migrations.
Cumulative human impacts have depressed Columbia River salmon populations to the point that most are now listed as threatened or endangered under the US Endangered Species Act (see http://www.nwr.noaa.gov/ESA-Salmon-Listings/Salmon-Populations/index.cfm).
However, almost without exception evaluations of the effects of dams have focused on ecological/demographic consequences — for example, how many fish are killed by the hydropower system and how this affects population viability (Kareiva et al. 2000; Petrosky et al. 2001; McClure et al. 2003), and relatively little attention has been paid to evolutionary changes that the hydropower system is likely to elicit in salmon.
In this respect, the situation in the Columbia River parallels the more general lack of attention to evolutionary changes associated with major anthropogenic rearrangements of ecosystems globally. This is a potentially serious omission, since ecological changes as pervasive as those caused by the Columbia River hydropower system are certain to profoundly alter the selective regimes that salmon experience, and some sort of evolutionary response is expected. What is not clear is how extensive these evolutionary changes might be and what their consequences would be for long-term viability.
Consequences of Large Dams for Migratory Fish
In this study, we make a first attempt to examine evolutionary consequences of large dams for migratory fishes. It is a broad topic, so our treatment is necessarily somewhat superficial.
First, we describe ecological/environmental changes associated with the Columbia River hydropower system, focusing primarily on mainstem dams on the Columbia and Snake Rivers.
Next, we consider how these changes have altered selective regimes experienced by salmon and what types of evolutionary responses might be expected.
Finally, we discuss the evolutionary challenges faced by Columbia River salmon in the broader context of what is known about evolutionary processes in other species, identify evolutionary considerations that will be particularly important to consider in salmon conservation and management, and outline research that could help to resolve critical uncertainties and increase our understanding of this complex topic.
Synthesis and Conclusions
Two conclusions were readily apparent.
First, selective regimes experienced by Pacific salmon have been profoundly altered by the Columbia River hydropower system.
Second, there is a strong heritable component to many of the traits that would be expected to be under strong selection as a result of these environmental changes.
However, evaluating the actual evolutionary responses by salmon populations, and what this ultimately means for long-term sustainability, is much more complicated.
To begin to address these issues, we first review some general evolutionary considerations and then turn to discussing data for salmon.
Abundant empirical evidence documents the reality of contemporary microevolution in natural populations (including Pacific and Atlantic salmon), but very strong directional selection is seldom observed (Kingsolver et al. 2001; Kinnison & Hendry 2001). Merilä et al. (2001) discussed a number of reasons why this might be the case. First, perhaps no evolution has actually occurred. Heritability of the trait(s) under consideration might be overestimated, or selection might be acting on a nonheritable part of the phenotype. Second, evolution might be occurring but its action masked by confounding factors. For example, because of genetic correlations among selected traits, in some cases evolution of bill size in Galapagos finches occurred in the opposite direction to that predicted (Grant & Grant 1995). Only a few studies (e.g. Hard 2004) have measured genetic correlations among life-history traits in Pacific salmon. Alternatively, the species might have evolved (changed its norm of reaction) just to maintain the same phenotype in an altered environment. Or, selection might be variable in space and/or time, so that the net evolutionary response is modest. Finally, statistical power might be insufficient to detect any but the most pronounced evolutionary changes.
We can conclude that the evolutionary responses of Pacific salmon to ecosystem changes caused by the Columbia River hydropower system will be difficult to predict and challenging to measure. For example, several different factors exert selective pressures for juvenile migration timing (growth rates; predation pressure in freshwater; marine conditions), and these factors do not necessarily all act in the same direction. This might help explain the apparent disconnect between the optimal time of arrival at the estuary of juvenile Chinook salmon and the observed time (Fig. 1).
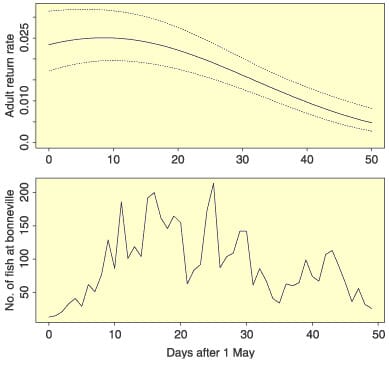
Fig. 1 Top: probability of adult return to Lower Granite Dam vs. juvenile arrival date to the estuary (below Bonneville Dam) for Snake River spring/summer Chinook salmon. Solid line is logistic regression; dotted lines are 95% confidence intervals. Analysis based on unpublished data for 4538 juveniles tagged with unique codes in 2002. Bottom: distribution of arrival time at Bonneville Dam in 2002 of tagged juveniles representative of the population as a whole.
In spite of apparently strong selection for early migration timing, these populations might find it difficult to compensate for dam-related delays by an evolutionary response. Attaining a critical size appears to be a trigger for initiation of seaward migration (Achord et al. 2007), and there is evidence that larger size at migration is also strongly selected for (Zabel & Williams 2002). Thus, an evolutionary shift to earlier migration timing might also require evolution of a higher growth rate. Although growth rate in Chinook salmon is heritable (h2 = 0.3; Hard 2004), increasing growth rates typically involve trade-offs with other fitness-related traits (Conover & Schultz 1997; Hard 2004).
Dams have their most dramatic and direct effects on the juvenile and adult migratory phases; in both cases, genotypes favoured under current conditions differ considerably from those favoured in pristine river systems. However, we have also seen that, indirectly, dams can produce an evolutionary response that is expressed at a different life stage (e.g. by increasing the cost of migration, which reduces energy available for sexual selection and increases the relative fitness of the nonanadromous life history). These general themes are broadly relevant to investigations of the evolutionary effects of dams on migratory species on a global scale.
An unusual, local twist is that current operation of the Columbia River hydropower system ensures that each year, some juveniles migrate downstream through all the dams, while others from the same population are transported in trucks or barges. As we have seen, the two groups of fish have different mortality rates while in the rivers and, presumably, during their early ocean residence. This repeated partitioning of a single population into components with different demographic trajectories is analogous to what occurs in a natural population subjected to hatchery supplementation (Waples & Drake 2004). Under these conditions, it can be very challenging to determine the net effects of selection within the population. A related phenomenon (and additional complexity) is that ecological changes create novel selective pressures not only among individuals within populations, but also among populations within species.
Although this topic is challenging and has been relatively neglected for a long time, a number of things can be done to help advance our understanding of the evolutionary responses of Pacific salmon to anthropogenic chan-ges, including those associated with the Columbia River hydropower system. Estimates of heritabilities can be obtained for more traits in more species, if possible in natural populations using modern molecular methods of parentage analysis. The potential upward bias in estimated heritabilities noted by Merila et al. (2001) can be addressed in part by more fully accounting for maternal effects in quantitative genetic studies.
Experiments to elucidate the genetic architecture (gene–gene correlations) of the target species are important to fully understand the effects of natural selection, which (except in simple computer models) seldom operates on a single trait. However, these evaluations can be complicated by evolution of the genetic covariance matrix itself. The evolutionary response of salmon to environmental change cannot be understood without also understanding the capacity of the species for phenotypic plasticity (expression of multiple phenotypes with the same genotype). The range of phenotypes expressed is known as the norm of reaction (Hutchings 2004), and reaction norms can be characterized by common-garden experiments. All of these approaches are challenging to implement with nonmodel species, especially those (such as Pacific salmon) that are age-structured, have a relatively long generation time (which typically equals or exceeds the experimental time available for a doctoral thesis), and spend half or more of their life in the highly unpredictable marine environment. Implementing some of these approaches, however, can provide vital information for conservation and management of migratory species such as salmon.
Climate change
Evidence is beginning to accumulate showing an evolutionary response by species to recent climate change (Bradshaw & Holzapfel 2006). Theoretical considerations and empirical results indicate that natural populations are capable of relatively rapid evolution (0.1–0.5 standard deviation of trait value per generation) over short time periods (tens or hundreds of generations), but the rate that natural populations can sustain for long periods of time is much lower (a few per cent standard deviation change in a trait per generation) (Kinnison & Hendry 2001).
Climate changes have important consequences for Pacific salmon and will interact synergistically with direct environmental effects of dams to determine the nature and extent of evolutionary responses by salmon. Some consequences are predictable in a general way (Mote et al. 2003): warming trends will shift precipitation toward winter rains rather than snow, and reduced snowmelt will produce earlier peak flows and less cool water in summer, when elevated stream temperatures can approach the thermal tolerance for salmon. Higher water temperatures will mean higher metabolic rates for juvenile salmon, as well as higher consumption rates for predators. Warmer thermal regimes might also promote the evolution and spread of infectious diseases, as well as the virulence of disease outbreaks.
In response to these new challenges, Columbia River salmon populations will have four options: (i) express a different phenotypic without any genetic change (phenotypic plasticity); (ii) evolve a different phenotype; (iii) move to a new location more compatible with their existing phenotype and life history; or (iv) go extinct.
We know that salmon can express plasticity for key life-history traits (Beckman & Dickhoff 1998), are capable of relatively rapid evolution in novel environments (Quinn et al. 2001), and can in some cases rapidly colonize newly accessible habitat (Milner 1987). We also know that salmon populations can go extinct — the fate of perhaps 30% of historic populations in the contiguous USA. (Gustafson et al. 2007). However, we still have a lot to learn before we have a sound basis for predicting which of these scenarios is most likely in any given circumstance.
Sustainability
A key question for management and conservation is, 'What do evolutionary changes associated with the hydropower system mean for long-term sustainability of salmon in the Columbia River?' Some general insights into this complex question can be gained by simple models of populations challenged by environmental changes. Because adaptation requires genetic deaths that result from the action of natural selection, populations experiencing rapid environmental changes often are in a race to adapt before they go extinct (Burger & Lynch 1995; Gomulkiewicz & Holt 1995). These studies show that the mean population phenotype typically evolves in parallel with, but lags behind, the optimum, and the magnitude of the lag is a crucial factor determining extinction risk. Figure 1 provides evidence of a lag of this type in timing of arrival of juvenile Chinook salmon to the estuary. In addition, random demographic and genetic events considerably increase extinction risk in small populations, so populations that have been reduced in size by anthropogenic factors are particularly vulnerable when challenged by rapid environmental changes. Both of these factors constrain the maximum sustained rate of evolution that a population can withstand. For Pacific salmon, two additional issues are particularly important for sustainability.
Biocomplexity
Although it is axiomatic that biocomplexity contributes to sustainability, empirical demonstrations of this ecological principle in natural systems are rare. Perhaps the best example involves sockeye salmon in Bristol Bay, Alaska, which have supported harvests on the order of 10 million fish per year for over a century, in spite of large changes in the proportional contribution of different stock complexes to the harvest (Hilborn et al. 2003). That is, overall abundance and productivity is more robust than any of the contributing components. Over longer time frames, the rich store of adaptive diversity expressed by Bristol Bay sockeye salmon provides the raw material upon which future evolution can act and the resilience to meet future environmental challenges. Impassable dams have already extirpated many salmon populations and life history types in the Columbia River basin (Bottom et al. 2005; Gustafson et al. 2007). If current conditions associated with hydropower development select for a narrower range of phenotypes than what has occurred historically, biocomplexity (and prospects for long-term sustainability) will be reduced. More study is needed to determine to what extent this might be true.
Darwinian debt
Evidence that fish populations have evolved maladaptive traits in response to size-selective harvest has led to the concept of a Darwinian debt, which must be repaid by further evolution before the population can restore its lost fitness (Walsh et al. 2006). This concept is also more broadly relevant to conservation of salmon in the Columbia River basin. Although it is difficult to predict the exact nature of evolutionary responses by salmon to altered environments, such responses are inevitable given the profound changes to selective regimes and mortality profiles. Even dams as massive as those in the Columbia River have a finite useful life of only a century or two, which represents the blink of an eye on evolutionary and geological timescales.
What might be the evolutionary consequences for salmon of returning the Columbia and Snake Rivers to more natural conditions (Williams 2006)? This would presumably restore selective regimes to something approaching historical patterns. However, to the extent that salmon have adapted to the radically altered ecological conditions of the current hydropower system, we might expect a rocky period of adjustment during which the populations have to repay the Darwinian debt they accrued by re-evolving adaptations to the restored natural conditions. Juveniles adapted to surviving spillways, turbines, diversion channels, and transportation in barges would have to re-evolve skills to navigate down the mainstem Columbia River at peak flows. Adults selected for the ability to navigate fish ladders and migrate through slack-water reservoirs might suddenly find they had insufficient energy reserves to migrate hundreds of kilometres upstream against strong currents and insufficient strength to surmount the renascent Celilo Falls. Timing of migration and other key life- history traits that had evolved in response to hydropower changes might be maladaptive in the new (old) environments. Thus, it is reasonable to expect that at least temporary reductions in fitness due to a Darwinian debt would accompany restoration of normative river conditions. With luck, the immediate reductions in direct mortality associated with the hydropower system would more than offset the interest on the Darwinian debt. It is not hard to imagine, however, that at least some populations might find themselves in a desperate race to re-evolve historical traits before they go extinct.
Although we have focused on Pacific salmon in one major river basin in the western USA, we expect that similar ecological and evolutionary considerations apply to Atlantic salmon (Salmo salar) in eastern North America and Europe, where dams also are a common feature of river ecosystems. More broadly, migratory fishes are of particular conservation concern on a global scale (e.g. McDowall 1999). Understandably, most conservation attention has focused on the extremely depressed population sizes of many migratory species. However, our analyses suggest that the nature and scale of ecosystem changes associated with large dams has the potential to elicit strong evolutionary responses in affected populations, and it will be difficult to craft effective, long-term conservation strategies without a better understanding of these contemporary evolutionary processes.
Further Reading
![]() |
- | You can view the full report by clicking here. |
February 2008